What Does Fncs Stand For
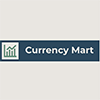
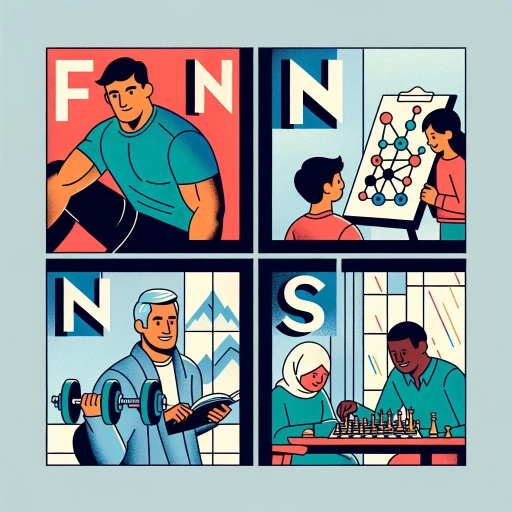
In the realm of advanced materials and technological innovation, the acronym FNCs has garnered significant attention for its potential to revolutionize various industries. Standing for Functional Nanocellulose, FNCs represent a cutting-edge development in the field of nanotechnology. This article delves into the multifaceted world of FNCs, beginning with an in-depth exploration of **Understanding the Acronym FNCs**, where we dissect the meaning and origins of this term. We then transition to **Technical Aspects of FNCs**, examining the intricate details of how these nanomaterials are engineered and their unique properties. Finally, we discuss **Real-World Applications and Impact**, highlighting how FNCs are transforming sectors such as biomedicine, sustainable packaging, and energy storage. By understanding the acronym, grasping the technical nuances, and appreciating the real-world applications, readers will gain a comprehensive insight into the transformative power of Functional Nanocellulose. Let us start by **Understanding the Acronym FNCs** to lay the foundation for this fascinating journey into the world of nanotechnology.
Understanding the Acronym FNCs
Understanding the acronym FNCs (Functional Nanocellulose) is crucial in today's technological and industrial landscape. This high-quality, informative, and engaging article delves into the multifaceted world of FNCs, providing a comprehensive overview that spans historical context, common uses, and industry-specific applications. Historically, the development of FNCs has been a gradual process, influenced by advancements in materials science and nanotechnology. The historical context of FNCs sets the stage for understanding how these materials have evolved over time. Moving forward, the common uses of FNCs highlight their versatility and widespread adoption across various sectors. From biomedical applications to sustainable packaging solutions, FNCs have proven to be highly adaptable and beneficial. Furthermore, industry-specific applications reveal the specialized roles that FNCs play in fields such as textiles, electronics, and construction. By exploring these three key areas, this article aims to provide a thorough understanding of FNCs and their significance. Let us begin by examining the historical context of FNCs, which lays the foundation for their current and future impact.
Historical Context of FNCs
The historical context of FNCs, or Functional Nanocellulose, is deeply intertwined with the evolution of materials science and the quest for sustainable technologies. The journey begins in the early 20th century when cellulose, a natural polymer found in plant cell walls, was first isolated and studied. Initially, cellulose was used primarily in the production of paper and textiles. However, as environmental concerns and the need for sustainable materials grew, researchers began to explore its potential beyond traditional applications. In the mid-20th century, advancements in nanotechnology opened new avenues for cellulose research. Scientists discovered that by breaking down cellulose into its nanoscale components—such as nanofibrils and nanocrystals—they could create materials with unique properties like enhanced strength, transparency, and biocompatibility. This breakthrough led to the development of Functional Nanocellulose (FNCs), which could be tailored for various industrial uses. The 1990s and 2000s saw a significant surge in FNCs research, driven by increasing global awareness of environmental issues and the need for renewable resources. Researchers from diverse fields—materials science, chemistry, biology, and engineering—collaborated to develop FNCs that could replace synthetic polymers in packaging, biomedical applications, and even electronics. This interdisciplinary approach accelerated the development of FNCs, enabling them to be used in innovative products such as biodegradable packaging materials, wound dressings, and even components in electronic devices. One of the pivotal moments in the history of FNCs was the establishment of dedicated research centers and consortia focused on nanocellulose. These institutions facilitated international collaboration, shared resources, and accelerated the translation of laboratory findings into commercial products. For instance, initiatives like the European Union's Horizon 2020 program and the U.S. Department of Agriculture's Forest Products Laboratory have played crucial roles in funding and coordinating FNCs research. Today, FNCs are at the forefront of sustainable material innovation. They offer a promising solution to reduce our reliance on fossil fuels and mitigate environmental impacts associated with traditional materials. The versatility of FNCs has led to their integration into various sectors, from consumer goods to advanced technologies. As research continues to advance, we can expect FNCs to play an increasingly significant role in shaping a more sustainable future. Understanding this historical context is essential for appreciating the full potential of FNCs. It highlights how scientific discovery, technological innovation, and collaborative efforts have transformed a natural polymer into a versatile tool for addressing some of the world's most pressing challenges. As we move forward, the continued development and application of FNCs will likely be a key factor in driving sustainable development and reducing our ecological footprint.
Common Uses of FNCs
**Common Uses of FNCs** Functional Nanocellulose (FNCs) has emerged as a versatile and sustainable material with a wide range of applications across various industries. One of the primary uses of FNCs is in the development of advanced biomaterials. Due to their unique properties such as high strength, biodegradability, and biocompatibility, FNCs are being explored for medical applications including wound dressings, tissue engineering scaffolds, and drug delivery systems. For instance, nanocellulose-based hydrogels can provide a moist environment that promotes wound healing while also offering antimicrobial properties. In the field of packaging, FNCs are gaining traction as an eco-friendly alternative to traditional plastics. Nanocellulose films and coatings can enhance the barrier properties of packaging materials, reducing the permeability to oxygen and moisture, thereby extending the shelf life of food products. This not only helps in reducing food waste but also minimizes the environmental impact associated with plastic packaging. The construction industry is another significant beneficiary of FNCs. Nanocellulose can be incorporated into building materials to improve their mechanical strength and thermal insulation properties. For example, nanocellulose-reinforced cement composites exhibit enhanced durability and resistance to cracking, making them ideal for sustainable building solutions. Additionally, FNCs are being utilized in the production of advanced paper products. By adding nanocellulose to paper pulp, manufacturers can create papers with improved tensile strength, opacity, and printability. This makes them suitable for high-quality printing applications such as packaging labels and specialty papers. In the realm of electronics, FNCs are being explored for their potential in creating flexible and transparent displays. Nanocellulose-based substrates offer a biodegradable alternative to traditional plastics used in display technology, aligning with the growing demand for sustainable electronics. Moreover, FNCs have shown promise in water treatment processes. Their high surface area and reactivity make them effective adsorbents for removing heavy metals and other contaminants from water. This application is particularly significant given the global need for clean water resources. Lastly, the cosmetic industry is leveraging the benefits of FNCs in skincare products. Nanocellulose-based formulations can provide moisturizing effects while also offering anti-aging benefits due to their ability to form a protective barrier on the skin's surface. In summary, the versatility of FNCs spans multiple sectors, from healthcare and packaging to construction and electronics, highlighting their potential as a key component in sustainable innovation across various industries.
Industry-Specific Applications
In the realm of industry-specific applications, Functional Nanocellulose (FNCs) emerges as a versatile and innovative material with far-reaching potential. FNCs, derived from cellulose—a naturally abundant polymer found in plant cell walls—undergo various chemical and physical modifications to enhance their properties. These tailored modifications enable FNCs to cater to diverse industrial needs, making them a cornerstone in multiple sectors. ### Biomedical Applications In the biomedical field, FNCs are being explored for their biocompatibility and biodegradability. They are used in wound healing dressings due to their ability to promote tissue regeneration and manage moisture effectively. Additionally, FNCs are incorporated into drug delivery systems because of their controlled release capabilities, ensuring that therapeutic agents are delivered precisely where needed. Their non-toxic nature also makes them suitable for implantable devices and tissue engineering scaffolds. ### Packaging and Food Industry The packaging sector benefits significantly from FNCs due to their barrier properties against oxygen, water vapor, and other gases. This makes them ideal for creating sustainable packaging solutions that extend the shelf life of food products while reducing the reliance on non-biodegradable plastics. Furthermore, FNCs can be used as edible films or coatings for fruits and vegetables, enhancing their freshness and safety. ### Construction and Building Materials In construction, FNCs are utilized to improve the performance of building materials. They can be added to concrete mixtures to enhance strength, durability, and thermal insulation properties. This not only reduces the environmental impact of traditional construction materials but also improves the energy efficiency of buildings. Moreover, FNCs can be used in the production of sustainable composites for various architectural applications. ### Electronics and Energy Storage The electronics industry is also leveraging FNCs for their unique electrical properties. They are being researched as potential components in flexible electronics, such as wearable devices and flexible displays, due to their mechanical strength and electrical conductivity. In energy storage, FNCs are explored as sustainable alternatives in battery technologies like supercapacitors and lithium-ion batteries, offering improved performance and reduced environmental footprint. ### Textiles and Fashion In the textile industry, FNCs are used to create sustainable fabrics with enhanced properties. They can improve the strength, softness, and moisture-wicking abilities of fabrics while maintaining breathability. This makes them particularly appealing for sportswear and technical textiles where performance is crucial. Additionally, FNCs can be integrated into smart fabrics that monitor health parameters or provide UV protection. ### Environmental Remediation FNCs also play a significant role in environmental remediation efforts. Their high surface area and reactivity make them effective in adsorbing pollutants from water and air. This capability is particularly valuable in cleaning up industrial effluents and contaminated soil, contributing to a cleaner environment. In summary, the versatility of FNCs across various industries underscores their potential as a transformative material. From biomedical applications to sustainable packaging, construction materials, electronics, textiles, and environmental remediation, FNCs offer a wide range of benefits that align with global sustainability goals. Understanding the acronym FNCs is crucial for appreciating the breadth of their impact and the future possibilities they hold in shaping innovative solutions across multiple sectors.
Technical Aspects of FNCs
In the realm of advanced materials, Functional Nanocomposites (FNCs) stand out for their unique properties and versatile applications. Understanding the technical aspects of FNCs is crucial for harnessing their full potential. This article delves into three key areas: **Definition and Structure**, **Functional Components**, and **Operational Mechanisms**. First, we will explore the **Definition and Structure** of FNCs, which lays the foundational understanding of what constitutes these materials and how their structural elements contribute to their overall performance. This section will clarify the terminology and provide a detailed look at the composition and arrangement of FNCs. Next, we will examine the **Functional Components** that make up FNCs, highlighting the specific roles each component plays in enhancing the material's functionality. This includes discussions on the integration of various nanomaterials and how they interact to achieve desired properties. Finally, we will delve into the **Operational Mechanisms** that govern how FNCs work, explaining the underlying principles and processes that enable their unique functionalities. By understanding these mechanisms, researchers and engineers can better design and optimize FNCs for specific applications. To begin, let's start with the fundamental **Definition and Structure** of FNCs, which sets the stage for a comprehensive understanding of these advanced materials.
Definition and Structure
**Definition and Structure** Functional Nanocellulose (FNCs) represents a cutting-edge domain within materials science, where the inherent properties of cellulose are harnessed and enhanced at the nanoscale. Cellulose, a biopolymer found abundantly in plant cell walls, is transformed into FNCs through various mechanical, chemical, or enzymatic treatments that break down its fibrous structure into nanofibrils or nanoparticles. This process not only retains the biodegradable and renewable nature of cellulose but also amplifies its mechanical strength, thermal stability, and surface area, making it an ideal candidate for diverse applications. The structure of FNCs is characterized by their dimensions and morphology. Typically, nanocellulose can be categorized into three main forms: Cellulose Nanofibrils (CNFs), Cellulose Nanocrystals (CNCs), and Bacterial Cellulose (BC). CNFs are long, flexible fibrils with diameters ranging from 3 to 100 nanometers and lengths up to several micrometers. These fibrils are often produced through mechanical disintegration methods such as grinding or high-pressure homogenization. On the other hand, CNCs are rod-like crystals with diameters between 5 to 20 nanometers and lengths from 100 to 500 nanometers, obtained primarily through acid hydrolysis which removes amorphous regions of cellulose. BC, produced by certain bacteria like *Gluconacetobacter xylinus*, forms a network of nanofibrils that can be harvested directly from the culture medium. The unique structure of FNCs confers them with exceptional properties such as high tensile strength, Young's modulus, and aspect ratio, making them suitable for reinforcing polymers in composite materials. Additionally, their large surface area and hydrophilic nature facilitate interactions with other materials at the molecular level, enhancing their potential in drug delivery systems, tissue engineering scaffolds, and even as sustainable alternatives in packaging materials. Incorporating FNCs into various matrices can significantly improve the mechanical performance and durability of the resulting composites. For instance, when integrated into biodegradable polymers like polylactic acid (PLA) or polyhydroxyalkanoates (PHA), FNCs can enhance the stiffness and toughness of these materials without compromising their biodegradability. This synergy opens up new avenues for developing sustainable products that meet both environmental and performance criteria. Moreover, the surface chemistry of FNCs can be tailored through chemical modifications such as carboxylation, sulfation, or grafting with functional groups. These modifications enable FNCs to interact specifically with target molecules or cells, thereby expanding their utility in biomedical applications like wound healing dressings and biosensors. In summary, the definition and structure of FNCs underscore their versatility and potential across multiple disciplines. By understanding and manipulating the nanoscale architecture of cellulose, researchers can unlock a wide range of innovative applications that leverage the inherent strengths of this renewable biopolymer while addressing pressing environmental concerns. As research continues to evolve in this field, it is clear that FNCs will play a pivotal role in shaping sustainable technologies for the future.
Functional Components
**Functional Components (FNCs)** are a cornerstone of modern software development, particularly in the realm of React, a popular JavaScript library for building user interfaces. FNCs stand for Functional Components, which are a type of component that leverages the power of functional programming principles to simplify and streamline the development process. In the context of React, Functional Components are defined as pure functions that take in props and return JSX elements. Unlike Class Components, which are based on ES6 classes and require the use of lifecycle methods, FNCs are more straightforward and easier to manage. They do not have their own state or lifecycle methods; instead, they rely on hooks to handle state and side effects. This simplicity makes them highly maintainable and easier to test. One of the key technical aspects of FNCs is their use of **hooks**. Introduced in React 16.8, hooks allow developers to "hook into" React state and lifecycle methods from functional components. The most commonly used hooks include `useState` for managing state, `useEffect` for handling side effects, and `useContext` for accessing context (shared state). These hooks enable developers to write more concise and readable code by eliminating the need for complex class-based logic. Another significant advantage of FNCs is their **performance**. Since they do not have the overhead of classes and lifecycle methods, they are generally faster and more efficient. This is particularly important in applications where performance is critical, such as real-time data visualization or high-traffic web applications. **Memoization** is another technical aspect that plays a crucial role in optimizing FNCs. Memoization involves caching the results of expensive function calls so that they can be reused when the same inputs occur again. In React, this can be achieved using the `useMemo` hook, which helps in preventing unnecessary re-renders and improving overall application performance. Moreover, FNCs promote a **declarative programming style**, which aligns well with the principles of React. Declarative programming focuses on specifying what you want to achieve rather than how to achieve it. This approach makes it easier for developers to reason about their code and reduces the likelihood of bugs. In terms of **best practices**, it's important to keep FNCs small and focused on a single task. This modular approach ensures that components are reusable and easier to maintain. Additionally, using tools like ESLint with React-specific plugins can help enforce coding standards and catch potential issues early in the development cycle. Overall, Functional Components offer a powerful and efficient way to build robust and scalable user interfaces in React. Their simplicity, combined with the versatility of hooks and other technical features, makes them an indispensable tool for modern web development. As the landscape of front-end development continues to evolve, understanding and mastering FNCs will remain essential for any developer looking to create high-quality, performant applications.
Operational Mechanisms
**Operational Mechanisms** Operational mechanisms are the core components that enable Functional Nanocellulose (FNCs) to perform their intended functions efficiently. FNCs, which stand for Functional Nanocellulose, are engineered nanomaterials derived from cellulose, a natural polymer found in plant cell walls. The operational mechanisms of FNCs involve several key aspects that contribute to their versatility and effectiveness in various applications. 1. **Surface Modification**: One of the primary operational mechanisms of FNCs is surface modification. This process involves chemically or physically altering the surface properties of nanocellulose to enhance its interaction with other materials or to introduce specific functionalities. For instance, grafting functional groups such as carboxyl, hydroxyl, or amino groups onto the nanocellulose surface can improve its compatibility with polymers, metals, or biological systems, thereby expanding its range of applications. 2. **Dispersion and Stability**: The ability of FNCs to disperse uniformly in different media is crucial for their performance. Operational mechanisms here include the use of surfactants, sonication, or high-shear mixing to ensure stable dispersion. This uniform dispersion is essential for applications such as nanocomposites, where homogeneous distribution of FNCs within a matrix material can significantly enhance mechanical properties. 3. **Interfacial Interactions**: The interfacial interactions between FNCs and other components in a system are another critical operational mechanism. These interactions can be tailored through surface modification to achieve specific outcomes, such as improved adhesion in composite materials or enhanced biocompatibility in biomedical applications. Understanding and controlling these interactions at the molecular level allows for the optimization of FNC performance. 4. **Self-Assembly**: Self-assembly is a spontaneous process where FNCs organize into ordered structures without external direction. This operational mechanism leverages the inherent properties of nanocellulose, such as its hydrophilicity and hydrogen bonding capabilities, to form complex architectures like hydrogels, aerogels, or nanopapers. Self-assembly is particularly useful in creating materials with unique properties like high porosity, mechanical strength, and biodegradability. 5. **Biodegradability and Sustainability**: The biodegradable nature of FNCs is an important operational mechanism, especially in environmental and biomedical contexts. Cellulose-based materials can be designed to degrade under specific conditions, such as exposure to enzymes or certain pH levels, making them ideal for applications where sustainability and minimal environmental impact are paramount. 6. **Scalability and Processability**: Finally, the operational mechanisms of FNCs must also consider scalability and processability. Techniques such as mechanical grinding, enzymatic treatment, and ionic liquid processing are employed to produce FNCs on a large scale while maintaining their structural integrity and functional properties. Efficient processing methods ensure that FNCs can be integrated into various industrial workflows without compromising their performance. In summary, the operational mechanisms of FNCs are multifaceted and involve careful manipulation of surface properties, dispersion, interfacial interactions, self-assembly, biodegradability, and scalability. By understanding and optimizing these mechanisms, researchers and engineers can unlock the full potential of FNCs across diverse fields, from advanced materials science to biomedical engineering and sustainable technologies.
Real-World Applications and Impact
The real-world applications and impact of advanced technologies are multifaceted and far-reaching, influencing various sectors in profound ways. At the heart of these applications are three key areas: Industrial and Commercial Uses, Scientific and Research Applications, and Societal and Environmental Impact. In the industrial and commercial sphere, cutting-edge technologies drive efficiency, innovation, and competitiveness. For instance, automation and artificial intelligence are transforming manufacturing processes, enabling faster production and higher quality outputs. In the realm of scientific and research applications, these technologies facilitate groundbreaking discoveries and advancements. High-performance computing, for example, allows scientists to simulate complex phenomena and analyze vast datasets, leading to breakthroughs in fields such as medicine, climate science, and materials engineering. Moreover, the societal and environmental impact of these technologies cannot be overstated. They play a crucial role in addressing global challenges like climate change, public health, and sustainable development. From smart grids that optimize energy distribution to wearable devices that monitor health metrics, these innovations are reshaping our daily lives and contributing to a more sustainable future. Transitioning to the industrial and commercial uses, it is evident that these technologies are not just tools but game-changers. They redefine business models, enhance productivity, and open up new market opportunities. The integration of advanced technologies in industries such as logistics, finance, and healthcare is a prime example of how innovation can lead to significant economic and operational benefits. This article will delve deeper into these industrial and commercial uses, exploring how they are revolutionizing the way businesses operate and thrive.
Industrial and Commercial Uses
In the realm of industrial and commercial applications, the acronym FNCs (Functional Nanocellulose) stands out as a transformative material with far-reaching implications. Functional Nanocellulose, derived from cellulose fibers, is engineered at the nanoscale to enhance its mechanical, thermal, and optical properties. This innovative material has revolutionized various sectors by offering sustainable, biodegradable, and highly versatile solutions. ### Industrial Applications 1. **Construction and Building Materials**: FNCs are being integrated into construction materials to improve strength, durability, and thermal insulation. When added to concrete, FNCs can reduce the need for cement while enhancing the overall structural integrity of buildings. This not only reduces environmental impact but also lowers construction costs. 2. **Packaging**: The packaging industry is adopting FNCs to create biodegradable and compostable packaging solutions. These materials replace traditional plastics, significantly reducing plastic waste and contributing to a more sustainable future. 3. **Textiles**: In textile manufacturing, FNCs are used to create high-performance fabrics with enhanced strength, moisture-wicking properties, and UV resistance. This makes them ideal for applications ranging from sportswear to medical textiles. 4. **Paper and Pulp**: The paper industry benefits from FNCs by improving the strength and printability of paper products. This results in higher quality prints and reduced material usage, making the production process more efficient. ### Commercial Applications 1. **Consumer Goods**: FNCs are finding their way into consumer goods such as cosmetics, personal care products, and food packaging. For instance, in cosmetics, FNCs can act as natural thickeners and stabilizers, replacing synthetic alternatives. 2. **Automotive**: The automotive sector is exploring the use of FNCs in composite materials for vehicle parts. These composites offer lighter weight without compromising on strength, which can lead to improved fuel efficiency and reduced emissions. 3. **Medical Devices**: In healthcare, FNCs are being used in wound dressings due to their biocompatibility and ability to promote wound healing. They also serve as scaffolds in tissue engineering applications. 4. **Electronics**: Researchers are investigating the potential of FNCs in electronic devices such as flexible displays and sensors. The unique properties of FNCs make them suitable for creating flexible yet durable electronic components. ### Impact The integration of FNCs across these industries has a profound impact on sustainability and innovation: - **Sustainability**: By leveraging a renewable resource like cellulose, industries can significantly reduce their carbon footprint and reliance on non-renewable materials. - **Innovation**: The versatility of FNCs opens up new avenues for product development, enabling companies to create novel products with enhanced performance characteristics. - **Economic Benefits**: The use of FNCs can lead to cost savings through reduced material usage and improved product lifetimes, contributing positively to economic growth. In conclusion, Functional Nanocellulose is redefining industrial and commercial landscapes by offering a sustainable, high-performance alternative to traditional materials. Its diverse applications underscore its potential to drive innovation while promoting environmental stewardship. As research continues to advance the properties and uses of FNCs, it is clear that this material will play a pivotal role in shaping the future of various industries.
Scientific and Research Applications
**Scientific and Research Applications** In the realm of scientific and research applications, the acronym "FNCS" stands for "Framework for Network Co-Simulation," a powerful tool that has revolutionized the way researchers and scientists conduct simulations across various disciplines. FNCS is designed to facilitate the integration of multiple simulation tools and models, enabling a more comprehensive and accurate analysis of complex systems. This framework is particularly valuable in fields such as power systems engineering, transportation, and environmental science, where understanding the interactions between different components is crucial. For instance, in power systems engineering, FNCS allows researchers to simulate the behavior of electrical grids under various conditions, including the integration of renewable energy sources and the impact of smart grid technologies. By co-simulating different models—such as those for power flow, control systems, and communication networks—researchers can predict potential issues and optimize grid performance. Similarly, in transportation research, FNCS can be used to simulate traffic flow and the impact of autonomous vehicles on urban mobility, helping to design more efficient and safe transportation systems. In environmental science, FNCS enables the co-simulation of climate models, hydrological systems, and ecological models to study the interconnectedness of environmental processes. This capability is essential for predicting the effects of climate change and developing strategies for sustainable resource management. The flexibility of FNCS also extends to other areas such as healthcare, where it can be used to model the spread of diseases and evaluate the effectiveness of public health interventions. The impact of FNCS is not limited to academic research; it also has significant practical applications. For example, utility companies can use FNCS to test and validate new grid management strategies before implementing them in real-world scenarios, reducing the risk of system failures and improving overall reliability. Similarly, urban planners can utilize FNCS to design smarter cities by simulating the effects of different infrastructure developments on traffic flow and energy consumption. Moreover, FNCS fosters collaboration among researchers from diverse backgrounds by providing a common platform for integrating their models. This interdisciplinary approach accelerates innovation and leads to more robust and reliable research outcomes. The open-source nature of FNCS further democratizes access to advanced simulation capabilities, making it a valuable resource for both established researchers and emerging scientists. In summary, FNCS is a versatile and powerful tool that enhances the capabilities of scientific and research applications across multiple fields. Its ability to integrate various models and simulate complex systems makes it an indispensable asset for advancing our understanding of the world and solving real-world problems. As research continues to evolve, the role of FNCS will only become more pivotal in driving innovation and improving the accuracy of scientific simulations.
Societal and Environmental Impact
The societal and environmental impact of technological advancements, such as those represented by the acronym FNCs (Functional Nanocomposites), is multifaceted and far-reaching. On the societal front, FNCs have the potential to revolutionize various sectors by enhancing performance, efficiency, and sustainability. For instance, in healthcare, FNCs can be used to develop advanced diagnostic tools and therapeutic agents, leading to improved patient outcomes and reduced healthcare costs. In the energy sector, these materials can enhance the efficiency of solar cells and fuel cells, contributing to a cleaner and more sustainable energy landscape. Additionally, FNCs can improve the durability and functionality of consumer products, from electronics to textiles, thereby reducing waste and extending product lifetimes. From an environmental perspective, the impact of FNCs is equally significant. By enabling the development of more efficient technologies, FNCs can help reduce energy consumption and greenhouse gas emissions. For example, advanced nanocomposite materials used in building insulation can significantly lower heating and cooling needs, thereby reducing energy consumption. Moreover, FNCs can play a crucial role in environmental remediation by creating more effective filters for water purification and air pollution control. The use of biodegradable nanocomposites in packaging materials could also mitigate plastic waste, a major environmental concern. Furthermore, the integration of FNCs into agricultural practices could lead to more sustainable farming methods, such as enhanced crop yields with reduced chemical usage. However, it is also important to consider the potential risks associated with the widespread adoption of FNCs. Ensuring the safe handling and disposal of these materials is critical to prevent unintended environmental harm. Regulatory frameworks and rigorous testing protocols must be established to safeguard both human health and the environment. Despite these challenges, the overall societal and environmental benefits of FNCs make them a promising area of research and development, offering substantial opportunities for improving quality of life while protecting the planet for future generations. By leveraging these advanced materials responsibly, we can foster a more sustainable and equitable society.