What Is 10 Degrees Celsius In Fahrenheit
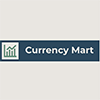
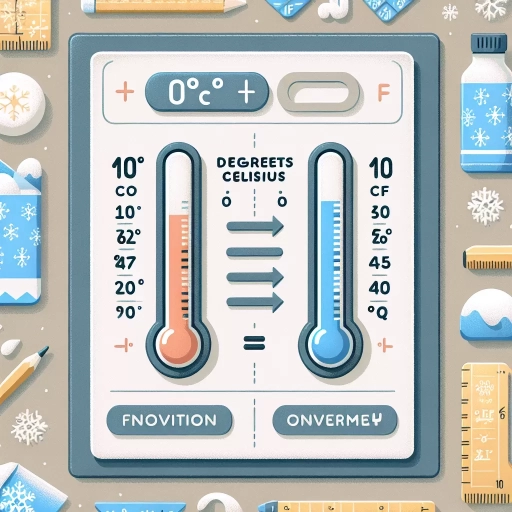
Understanding temperature conversions is a fundamental skill in various fields, from science and engineering to everyday life. One common query involves converting 10 degrees Celsius to Fahrenheit, a task that requires a basic grasp of thermometric scales and their interconversion. This article delves into the intricacies of this conversion, starting with **Understanding the Basics of Temperature Conversion**, where we explore the definitions and differences between Celsius and Fahrenheit scales. We then proceed to **The Mathematical Process of Converting Celsius to Fahrenheit**, detailing the step-by-step calculation necessary to transform 10 degrees Celsius into its Fahrenheit equivalent. Finally, we examine **Practical Applications and Real-World Examples**, highlighting scenarios where such conversions are crucial. By the end of this article, readers will not only know what 10 degrees Celsius is in Fahrenheit but also appreciate the broader context and utility of temperature conversion. Let's begin by laying the groundwork with an understanding of the basics.
Understanding the Basics of Temperature Conversion
Understanding the basics of temperature conversion is a fundamental skill that bridges various scientific and everyday applications. This article delves into the essential aspects of temperature measurement, providing a comprehensive overview that is both informative and engaging. We begin by exploring the **Introduction to Celsius and Fahrenheit Scales**, where we dissect the origins, definitions, and practical uses of these two primary temperature scales. This foundational knowledge is crucial for any further discussion on temperature conversion. To contextualize these scales, we also examine the **Historical Context of Temperature Measurement**, tracing the evolution of temperature measurement from ancient civilizations to modern times. This historical perspective highlights the development of thermometry and the contributions of key figures who shaped our current understanding of temperature. Finally, we emphasize the **Importance of Accurate Temperature Conversion**, illustrating how precise temperature measurements impact fields such as science, engineering, and even daily life. Accurate conversions are vital for ensuring safety, efficiency, and reliability in various applications. By grasping these concepts, readers will gain a solid understanding of temperature conversion, starting with a clear comprehension of the Celsius and Fahrenheit scales. Let us begin this journey by diving into the **Introduction to Celsius and Fahrenheit Scales**.
Introduction to Celsius and Fahrenheit Scales
Understanding the basics of temperature conversion begins with a solid grasp of the two most commonly used temperature scales: Celsius and Fahrenheit. The Celsius scale, named after Swedish astronomer Anders Celsius, is widely used in scientific and everyday applications around the world. It defines 0 degrees Celsius as the freezing point of water and 100 degrees Celsius as the boiling point, making it a straightforward and intuitive system. On the other hand, the Fahrenheit scale, developed by German physicist Gabriel Fahrenheit, is predominantly used in the United States. It sets 32 degrees Fahrenheit as the freezing point of water and 212 degrees Fahrenheit as the boiling point. This scale has a more complex relationship between its reference points, which can make conversions slightly more challenging. The historical context of these scales is fascinating; while Celsius's system was introduced in the 18th century and quickly gained international acceptance due to its simplicity and logical structure, Fahrenheit's scale was developed earlier but has seen declining use globally. Despite this, understanding both scales is crucial for effective communication across different regions and fields. For instance, in meteorology, weather forecasts often use Celsius in most parts of the world but switch to Fahrenheit in the United States. Similarly, in scientific research, Celsius is the preferred unit due to its ease of calculation and consistency with other metric system measurements. To convert between these two scales, one must understand their mathematical relationship. The formula to convert Celsius to Fahrenheit is \( \text{F} = \frac{9}{5}C + 32 \), where \( C \) is the temperature in Celsius and \( F \) is the temperature in Fahrenheit. Conversely, to convert Fahrenheit to Celsius, the formula is \( C = \frac{5}{9}(F - 32) \). These formulas are essential tools for anyone needing to switch between the two scales, whether for everyday purposes or in professional settings. In practical terms, knowing these conversion formulas can be very useful. For example, if you are traveling from a country that uses Celsius to one that uses Fahrenheit, being able to convert temperatures can help you better understand weather forecasts or cooking instructions. Additionally, in scientific contexts where precise temperature measurements are critical, accurate conversions are vital for maintaining data integrity and ensuring consistent results. In conclusion, mastering the Celsius and Fahrenheit scales is fundamental for anyone looking to understand temperature conversion. By recognizing the historical background, practical applications, and mathematical relationships between these two scales, individuals can navigate temperature-related information with confidence. Whether you are a student learning about thermodynamics or a traveler adjusting to a new climate, having a clear understanding of both Celsius and Fahrenheit will enhance your ability to interpret and communicate temperature data effectively. This foundational knowledge not only aids in everyday life but also forms a crucial part of scientific literacy and global communication.
Historical Context of Temperature Measurement
The historical context of temperature measurement is a rich and evolving narrative that spans centuries, reflecting human ingenuity and the quest for precision. The earliest attempts at measuring temperature date back to ancient civilizations, where observations of thermal changes were often qualitative rather than quantitative. For instance, the Greek philosopher Aristotle noted that water expands when heated and contracts when cooled, laying foundational principles for later scientific inquiry. However, it wasn't until the 16th century that the first thermoscopes were developed, primarily by Italian scientists such as Santorio Santorio and Galileo Galilei. These early devices used air or water to indicate temperature changes but lacked a standardized scale. The breakthrough came in the 17th century with the invention of the first mercury thermometer by Italian physicist Ferdinando II de' Medici in 1654. This innovation allowed for more accurate and consistent measurements. Shortly thereafter, in 1665, English scientist Robert Hooke proposed the idea of a temperature scale based on the freezing and boiling points of water. However, it was not until the late 18th century that the modern temperature scales were established. In 1742, Swedish astronomer Anders Celsius introduced the Celsius scale, which defined 100 degrees as the difference between the freezing and boiling points of water. Around the same time, German physicist Gabriel Fahrenheit developed his own scale, which became widely used in English-speaking countries. Fahrenheit's scale, introduced in 1724, was initially based on a mixture of ice, water, and ammonium chloride for its zero point and the human body temperature for its 96-degree mark. Later adjustments standardized it to the familiar 32 degrees for freezing and 212 degrees for boiling water. The coexistence of these two scales has led to ongoing discussions about their relative merits and practical applications. While the Celsius scale is now globally accepted in scientific and most everyday contexts due to its simplicity and logical structure, Fahrenheit remains prevalent in certain regions like the United States. Understanding this historical context is crucial for appreciating the nuances of temperature conversion. The transition from one scale to another involves more than just arithmetic; it reflects a deeper understanding of how different societies have approached the measurement of thermal energy over time. For example, converting 10 degrees Celsius to Fahrenheit requires not only knowing the formula (°F = °C × 9/5 + 32) but also recognizing why such a conversion is necessary in the first place. This historical backdrop underscores that temperature measurement is not just a technical exercise but also a cultural and scientific journey that continues to shape our daily lives and scientific endeavors. In summary, the evolution of temperature measurement from qualitative observations to precise quantification has been a gradual process influenced by various scientific minds across history. The development of standardized scales by Celsius and Fahrenheit has facilitated global communication and consistency in thermal measurements. As we navigate between these scales today, we honor the legacy of those who pioneered our understanding of temperature while ensuring that our measurements remain accurate and meaningful in both practical and scientific contexts.
Importance of Accurate Temperature Conversion
Understanding the importance of accurate temperature conversion is crucial in various aspects of life, from everyday applications to critical scientific and industrial processes. Temperature conversion, particularly between Celsius and Fahrenheit, is not just a simple mathematical exercise but a vital skill that ensures precision and safety. In scientific research, accurate temperature readings are essential for reproducibility and reliability of experiments. For instance, in chemistry and physics, even a slight deviation in temperature can significantly alter the outcome of reactions or experiments, making precise conversions indispensable. In industrial settings, such as manufacturing and engineering, accurate temperature control is critical for maintaining product quality and ensuring safety standards. Incorrect temperature readings can lead to material defects, equipment failure, or even hazardous conditions. For example, in the aerospace industry, precise temperature control is vital for the integrity of materials used in aircraft components, where deviations could compromise safety. In healthcare, accurate temperature conversion is vital for diagnosing and treating patients. Medical professionals rely on precise body temperature readings to assess patient health, and any inaccuracies could lead to misdiagnosis or inappropriate treatment. Additionally, in pharmaceuticals, the storage and transportation of medications often require specific temperature ranges to maintain their efficacy and safety. In everyday life, understanding temperature conversion helps individuals make informed decisions about weather forecasting, cooking, and even home maintenance. For instance, knowing that 10 degrees Celsius is equivalent to 50 degrees Fahrenheit can help someone gauge whether it is suitable for outdoor activities or if their refrigerator is at the correct temperature. Moreover, global communication and collaboration rely heavily on standardized units of measurement. Since different countries use different temperature scales, accurate conversion ensures that information is communicated clearly and consistently across borders. This is particularly important in international trade, where specifications for products must be precise to avoid misunderstandings or compliance issues. In conclusion, the importance of accurate temperature conversion cannot be overstated. It underpins the reliability of scientific research, the safety and efficiency of industrial processes, the accuracy of medical diagnoses, and the clarity of global communication. By mastering the basics of temperature conversion, individuals can ensure precision in their work and daily lives, contributing to a more accurate and safe world.
The Mathematical Process of Converting Celsius to Fahrenheit
Converting Celsius to Fahrenheit is a fundamental mathematical process that is essential in various fields, including science, engineering, and everyday life. Understanding this conversion is crucial for accurate temperature readings and applications. This article delves into the intricacies of this process, providing a comprehensive guide that covers three key aspects: the formula for Celsius to Fahrenheit conversion, a step-by-step calculation example, and common mistakes to avoid during the conversion. The formula for converting Celsius to Fahrenheit is the cornerstone of this process, and it is imperative to grasp it accurately. This formula, \( \text{Fahrenheit} = (\text{Celsius} \times \frac{9}{5}) + 32 \), serves as the foundation for all conversions. By understanding and applying this formula correctly, one can ensure precise temperature conversions. To illustrate the practical application of this formula, we will provide a step-by-step calculation example. This example will walk readers through the conversion process, making it easier to visualize and replicate the steps in real-world scenarios. Additionally, we will highlight common mistakes to avoid during the conversion. These mistakes can often lead to inaccurate results, and being aware of them is vital for maintaining precision in temperature readings. By mastering these elements, individuals can confidently convert Celsius to Fahrenheit, ensuring accuracy and reliability in their calculations. Let us begin by exploring **The Formula for Celsius to Fahrenheit Conversion** in detail.
The Formula for Celsius to Fahrenheit Conversion
The formula for converting Celsius to Fahrenheit is a fundamental mathematical process that bridges the gap between two of the most commonly used temperature scales. This conversion is essential in various fields, including meteorology, chemistry, and everyday life, especially when dealing with international data or different measurement systems. The formula itself is straightforward yet precise: \( \text{Fahrenheit} = (\text{Celsius} \times \frac{9}{5}) + 32 \). This equation transforms the linear relationship between Celsius and Fahrenheit into a practical tool for quick and accurate conversions. To understand this process, it's crucial to break down the components of the formula. The multiplication by \( \frac{9}{5} \) adjusts the scale of Celsius to match the broader range of Fahrenheit, while adding 32 shifts the zero point to align with the freezing and boiling points of water in both scales. For instance, if you want to convert 10 degrees Celsius to Fahrenheit, you would apply the formula as follows: \( 10 \times \frac{9}{5} = 18 \), then add 32, resulting in \( 18 + 32 = 50 \) degrees Fahrenheit. This mathematical process is not just a simple arithmetic operation but also reflects the historical and scientific context behind these temperature scales. The Celsius scale, developed by Anders Celsius in 1742, is based on the freezing and boiling points of water at standard atmospheric pressure, with 0 degrees Celsius being the freezing point and 100 degrees Celsius being the boiling point. In contrast, the Fahrenheit scale, introduced by Gabriel Fahrenheit in 1724, has its own set of reference points, with 32 degrees Fahrenheit as the freezing point and 212 degrees Fahrenheit as the boiling point. Understanding this conversion formula is vital for various practical applications. For example, in weather forecasting, temperatures are often reported in Celsius in many parts of the world but need to be converted to Fahrenheit for audiences in countries like the United States. Similarly, in scientific research, precise temperature measurements are critical, and converting between scales ensures consistency and accuracy across different studies and datasets. In conclusion, the formula for converting Celsius to Fahrenheit is a simple yet powerful tool that facilitates communication and understanding across different measurement systems. By applying this formula, individuals can seamlessly transition between these two temperature scales, ensuring that data is accurately interpreted and applied in various contexts. Whether you are a scientist, a meteorologist, or simply someone interested in understanding temperature readings from around the world, mastering this conversion process is indispensable.
Step-by-Step Calculation Example
To illustrate the mathematical process of converting Celsius to Fahrenheit, let's delve into a step-by-step calculation example. This process is straightforward and involves a simple formula that can be applied universally. The formula to convert Celsius to Fahrenheit is given by \( F = \frac{9}{5}C + 32 \), where \( C \) is the temperature in Celsius and \( F \) is the temperature in Fahrenheit. ### Step-by-Step Calculation Example 1. **Identify the Given Temperature**: Suppose we want to convert 10 degrees Celsius to Fahrenheit. Here, \( C = 10 \). 2. **Apply the Formula**: Plug the value of \( C \) into the formula: \[ F = \frac{9}{5} \times 10 + 32 \] 3. **Perform Multiplication**: First, multiply \( \frac{9}{5} \) by 10: \[ \frac{9}{5} \times 10 = 18 \] 4. **Add 32**: Next, add 32 to the result of the multiplication: \[ 18 + 32 = 50 \] 5. **Final Result**: Therefore, 10 degrees Celsius is equal to 50 degrees Fahrenheit. This example demonstrates how the conversion formula works in practice. By following these steps, you can convert any temperature from Celsius to Fahrenheit with ease. The key is to ensure that each step of the calculation is performed accurately to obtain the correct result. This method can be applied to any given temperature in Celsius, making it a versatile tool for everyday use, whether in scientific applications, weather forecasting, or simply understanding temperature scales in different contexts. Understanding this process not only enhances your mathematical skills but also provides a practical tool for navigating different temperature scales effectively.
Common Mistakes to Avoid in Conversion
When converting Celsius to Fahrenheit, it is crucial to avoid common mistakes that can lead to inaccurate results. One of the most frequent errors is the misuse of the conversion formula. The correct formula to convert Celsius to Fahrenheit is \( F = \frac{9}{5}C + 32 \), where \( C \) is the temperature in Celsius and \( F \) is the temperature in Fahrenheit. Many people mistakenly apply this formula without properly converting the fraction or forgetting to add the constant 32, leading to significant discrepancies. Another common mistake is rounding too early in the calculation process. Precision is key when dealing with temperature conversions, especially in scientific or engineering contexts where small differences can be critical. Rounding intermediate results can accumulate errors, so it is advisable to carry out calculations with full precision until the final step. Additionally, confusion between the two scales can lead to incorrect interpretations. For instance, a temperature of 10 degrees Celsius might seem close to 10 degrees Fahrenheit due to the similar numerical value, but this is far from accurate. Understanding that the zero points and scaling factors of these two systems are different is essential for accurate conversions. Furthermore, using incorrect units or failing to label units properly can cause confusion and errors. Always ensure that the input and output temperatures are clearly labeled as Celsius or Fahrenheit to avoid mix-ups. In practical applications, such as cooking or weather forecasting, these mistakes can have real-world implications. For example, in cooking, a few degrees difference can affect the doneness of food significantly. In weather forecasting, accurate temperature conversions are vital for predicting weather patterns and issuing timely warnings. To illustrate this with a specific example, consider converting 10 degrees Celsius to Fahrenheit. Using the correct formula: \( F = \frac{9}{5}(10) + 32 = 18 + 32 = 50 \). If one were to round prematurely or forget the constant term, they might end up with an incorrect result like 18 degrees Fahrenheit instead of the correct 50 degrees Fahrenheit. In conclusion, avoiding these common mistakes—misusing the conversion formula, premature rounding, confusion between scales, incorrect unit usage, and lack of precision—is essential for accurate temperature conversions from Celsius to Fahrenheit. By being meticulous and understanding the nuances of each scale, you can ensure that your conversions are reliable and meaningful. This attention to detail is particularly important in various fields where temperature accuracy is paramount.
Practical Applications and Real-World Examples
In today's interconnected world, practical applications and real-world examples are crucial for understanding and leveraging various scientific and technological advancements. This article delves into three pivotal areas where these applications are particularly significant: Weather Forecasting and Climate Studies, Cooking and Food Preparation, and Scientific Research and Laboratory Settings. Each of these domains showcases how theoretical knowledge is translated into tangible benefits that impact our daily lives. For instance, in Weather Forecasting and Climate Studies, advanced meteorological tools and data analytics enable accurate predictions that save lives and protect infrastructure. In Cooking and Food Preparation, the application of chemistry and physics ensures safe and nutritious food production. Meanwhile, Scientific Research and Laboratory Settings rely on rigorous methodologies to develop new treatments, materials, and technologies. By exploring these practical applications, we can appreciate the direct impact of scientific inquiry on our world. Let us begin by examining how Weather Forecasting and Climate Studies have revolutionized our ability to predict and prepare for natural phenomena.
Weather Forecasting and Climate Studies
Weather forecasting and climate studies are pivotal in understanding and predicting the Earth's atmospheric conditions, which have profound impacts on various aspects of human life and the environment. These fields leverage advanced technologies, including satellite imaging, radar systems, and sophisticated computer models, to analyze atmospheric data and forecast weather patterns. For instance, numerical weather prediction (NWP) models use complex algorithms to process vast amounts of data from weather stations, buoys, and satellites to predict temperature, precipitation, wind patterns, and other meteorological phenomena. These forecasts are crucial for practical applications such as aviation, where accurate weather predictions ensure safe flight operations; agriculture, where farmers rely on weather forecasts to optimize planting and harvesting schedules; and emergency management, where timely warnings can save lives during severe weather events like hurricanes or tornadoes. Climate studies, on the other hand, focus on long-term trends and patterns in the Earth's climate system. By analyzing historical climate data and using climate models, scientists can understand how human activities such as greenhouse gas emissions are influencing global temperatures and weather patterns. This knowledge is essential for developing policies aimed at mitigating climate change and adapting to its impacts. For example, climate models have helped predict sea-level rise, which informs coastal planning and infrastructure development to protect communities from flooding. Additionally, climate studies support the development of renewable energy strategies by identifying regions with optimal conditions for solar or wind power generation. Real-world examples of the practical applications of weather forecasting and climate studies abound. In the realm of public health, accurate weather forecasts help predict heatwaves or cold snaps that can lead to increased mortality rates, allowing health authorities to take preventive measures. In the economic sector, weather forecasts are critical for industries like insurance, where accurate predictions of severe weather events help assess risk and set premiums. Furthermore, climate studies have been instrumental in guiding international agreements such as the Paris Agreement, which aims to limit global warming to well below 2 degrees Celsius above pre-industrial levels. In conclusion, weather forecasting and climate studies are not just academic pursuits but have significant real-world implications that touch every aspect of society. From ensuring public safety during extreme weather events to informing global policies on climate change, these fields are indispensable for navigating the complexities of our ever-changing environment. As technology continues to advance, the accuracy and utility of these forecasts will only improve, enabling more effective decision-making across various sectors and contributing to a more resilient and sustainable future.
Cooking and Food Preparation
Cooking and food preparation are not just essential life skills but also vibrant expressions of culture, creativity, and science. When we delve into the practical applications of cooking, we find a multitude of real-world examples that highlight its significance. For instance, understanding the thermal dynamics involved in cooking can help us appreciate why 10 degrees Celsius (50 degrees Fahrenheit) is a critical temperature in food safety. At this temperature, bacteria such as Salmonella and E. coli can multiply rapidly, making it crucial to either refrigerate or cook food promptly to prevent foodborne illnesses. This principle is particularly relevant in professional kitchens where precise temperature control is essential for maintaining food safety standards. In everyday cooking, the concept of temperature plays a pivotal role. For example, when baking a cake, achieving the right internal temperature ensures that the cake is fully cooked and safe to eat. Similarly, in sous vide cooking, precise temperature control allows for uniform cooking throughout the dish, resulting in consistent flavors and textures. These techniques are not just limited to professional chefs; home cooks can also benefit from understanding how different temperatures affect various ingredients. Moreover, the science behind cooking extends beyond temperature control. Chemical reactions such as the Maillard reaction, which occurs when amino acids and reducing sugars interact with heat, are responsible for the development of complex flavors and aromas in dishes like seared steaks or roasted vegetables. This reaction is a perfect example of how chemistry meets culinary art, enhancing the overall dining experience. In addition to these scientific aspects, cultural influences also play a significant role in cooking and food preparation. Traditional recipes often reflect historical and geographical contexts, making each dish a story about its origins. For instance, the use of specific spices in Indian curries or the technique of slow-cooking in French bouillabaisse are not just methods but also cultural narratives passed down through generations. Practical applications of cooking also extend into health and nutrition. Understanding how different cooking methods affect nutrient retention can help individuals make healthier choices. For example, steaming vegetables preserves more vitamins compared to boiling, while grilling meats can reduce fat content if done correctly. These insights are invaluable for those looking to maintain a balanced diet without compromising on flavor. Finally, the art of food preparation is deeply intertwined with sustainability and environmental consciousness. Techniques like meal planning, using leftovers creatively, and choosing locally sourced ingredients can significantly reduce food waste and support local economies. These practices not only benefit the environment but also foster a sense of community and resourcefulness in the kitchen. In conclusion, cooking and food preparation are multifaceted activities that blend science, culture, health, and sustainability. By understanding the practical applications and real-world examples of these elements, we can elevate our culinary skills, enhance our appreciation for different cuisines, and contribute positively to our communities and the environment. Whether it's ensuring food safety at 10 degrees Celsius or mastering the art of sous vide, each aspect of cooking offers a rich tapestry of knowledge and creativity waiting to be explored.
Scientific Research and Laboratory Settings
Scientific research and laboratory settings are the cornerstone of advancing our understanding of the world and solving real-world problems. These environments are meticulously designed to ensure precision, safety, and reproducibility, allowing scientists to conduct experiments that can be reliably repeated and verified. In a laboratory setting, researchers employ a variety of techniques and tools to collect data, analyze samples, and draw conclusions. For instance, in the field of chemistry, scientists might use spectroscopy to identify the molecular structure of compounds or chromatography to separate and analyze mixtures. Similarly, in biology labs, researchers may use microscopy to study cellular structures or genetic sequencing to understand the genetic makeup of organisms. The practical applications of scientific research conducted in these settings are vast and diverse. For example, medical research labs are crucial for developing new treatments and vaccines. The COVID-19 pandemic highlighted the importance of rapid scientific research, where labs around the world worked tirelessly to understand the virus, develop diagnostic tests, and create vaccines in record time. In environmental science, laboratory research helps monitor pollution levels, study climate change, and develop sustainable technologies. Industrial labs focus on improving manufacturing processes and creating new materials with enhanced properties. Real-world examples abound. In the field of materials science, lab research has led to the development of advanced materials like graphene and nanomaterials, which have applications in electronics, energy storage, and medical devices. Agricultural research labs work on improving crop yields and developing drought-resistant crops, which are essential for global food security. Moreover, forensic labs play a critical role in solving crimes by analyzing DNA evidence and other biological samples. The rigorous methodologies used in scientific research ensure that findings are reliable and can be translated into practical solutions. This involves strict adherence to protocols, meticulous record-keeping, and peer review processes to validate results. Additionally, collaboration between researchers from different disciplines often leads to innovative solutions that might not have been possible within a single field of study. In conclusion, scientific research and laboratory settings are essential for driving innovation and solving complex problems. The work conducted in these environments not only expands our knowledge but also has direct practical applications that improve our daily lives. Whether it's advancing medical treatments, enhancing environmental sustainability, or developing new technologies, the contributions of scientific research are invaluable and continue to shape our future.