What Colour Is Science
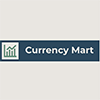
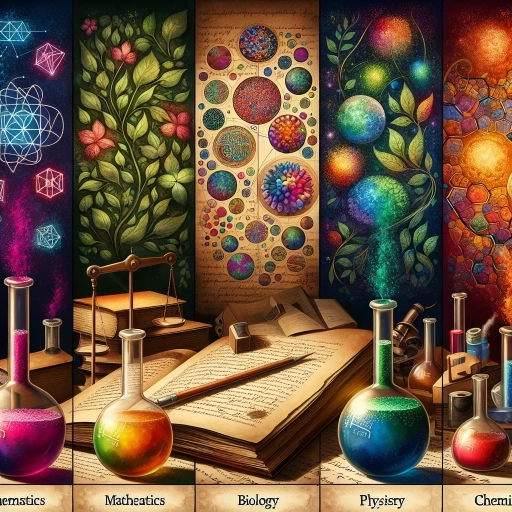
Color, often perceived as a subjective experience, is deeply rooted in the realm of science. It is a multifaceted phenomenon that not only captivates our senses but also plays a crucial role in various scientific disciplines. This article delves into the intricate world of color, exploring its scientific underpinnings, practical applications, and educational significance. We begin by **Understanding the Concept of Color in Science**, where we dissect the physical and biological mechanisms that define color perception. Next, we examine **Applications of Color in Various Scientific Fields**, highlighting how color is utilized in fields such as chemistry, biology, and physics to analyze, classify, and communicate data. Finally, we discuss **The Role of Color in Scientific Communication and Education**, illustrating how color enhances the clarity and engagement of scientific information. By grasping these aspects, we can appreciate the full spectrum of color's importance in science. Let us start our journey by understanding the fundamental concept of color in science.
Understanding the Concept of Color in Science
Understanding the concept of color in science is a multifaceted exploration that delves into various disciplines to provide a comprehensive view. At its core, color is a phenomenon that arises from the interaction of light, biological perception, and chemical properties. The physics of light and color lays the foundational framework, explaining how different wavelengths of light are absorbed, reflected, or transmitted by objects, resulting in the colors we see. This understanding is crucial for grasping the fundamental principles behind color production and manipulation. Additionally, the biological perception of color highlights how living organisms interpret these wavelengths through complex visual systems, influencing our subjective experience of color. Lastly, the chemical properties of pigments reveal the molecular structures responsible for absorbing and reflecting light, which is essential for understanding color in materials and dyes. By examining these three aspects—physics, biology, and chemistry—we can gain a deeper appreciation for the intricate mechanisms behind color. Let us begin by exploring the physics of light and color, which sets the stage for understanding how this fascinating phenomenon works.
1. The Physics of Light and Color
The physics of light and color is a fascinating realm that underpins our understanding of the visible world. At its core, light is a form of electromagnetic radiation, characterized by its wavelength and frequency. When light travels through a medium like air or water, it can be described in terms of its speed, direction, and intensity. The visible spectrum, which spans from approximately 380 nanometers (violet) to 780 nanometers (red), is the range of wavelengths that human eyes can detect. This spectrum is a small part of the broader electromagnetic spectrum, which includes forms of radiation such as ultraviolet (UV) and infrared (IR) that are invisible to us. Color arises from the interaction between light and matter. When light hits an object, some wavelengths are absorbed while others are reflected or transmitted. The reflected wavelengths are what we perceive as color. For instance, an object appears red because it reflects longer wavelengths (around 620-750 nanometers) and absorbs shorter ones. This principle is fundamental to understanding phenomena like pigmentation in biological organisms and the color properties of materials. The behavior of light also involves principles such as refraction, diffraction, and interference. Refraction occurs when light passes from one medium to another with a different optical density, causing it to bend; this is why objects appear distorted when partially submerged in water. Diffraction happens when light encounters an obstacle or a narrow opening, leading to the bending of light around edges or through small apertures. Interference occurs when two or more light waves overlap, resulting in patterns of constructive and destructive interference that can produce vivid displays like those seen in oil slicks or peacock feathers. In addition to these physical properties, the perception of color is also influenced by psychological and biological factors. Human vision relies on specialized cells in the retina called cones that are sensitive to different parts of the visible spectrum. There are three types of cones corresponding roughly to red, green, and blue light, allowing us to perceive a wide range of colors through additive color mixing. This biological basis for color perception explains why certain colors appear more vivid or harmonious to us and why some people may experience color vision deficiencies. Understanding the physics of light and color has numerous practical applications across various fields. In technology, it underpins the development of display devices like LEDs and LCDs, which rely on precise control over light emission and filtering to produce high-quality images. In art and design, knowledge of color theory helps artists create compositions that evoke specific emotions and moods by leveraging principles such as complementary colors and color harmony. In science itself, studying light and color helps us understand complex phenomena like atmospheric scattering (which explains why the sky appears blue) and the properties of materials at the molecular level. In conclusion, the physics of light and color is a rich and multifaceted subject that bridges fundamental scientific principles with everyday experiences. By delving into how light behaves and interacts with matter, we gain insights into both the physical world around us and our own perception of reality. This understanding not only enhances our appreciation for natural phenomena but also drives innovation across diverse disciplines.
2. Biological Perception of Color
**Biological Perception of Color** The biological perception of color is a complex and multifaceted process that involves the coordinated efforts of various components within the human visual system. At its core, color perception begins with the eye, where light enters through the cornea and is focused onto the retina by the lens. Here, specialized cells known as photoreceptors—rods and cones—convert light into electrical signals. Rods are sensitive to low light levels and are responsible for peripheral and night vision but do not mediate color vision. Cones, on the other hand, are responsible for color vision and are divided into three types: long-wavelength cones sensitive to red light, medium-wavelength cones sensitive to green light, and short-wavelength cones sensitive to blue light. When light of different wavelengths enters the eye, it stimulates these cone cells to varying degrees. The brain then interprets the relative stimulation of each type of cone to perceive different colors. This trichromatic theory of color vision was first proposed by Thomas Young and Hermann von Helmholtz in the 19th century and remains a cornerstone of our understanding of how we see colors. The signals from the photoreceptors are transmitted to bipolar cells, which relay them to ganglion cells that send the information via the optic nerve to the brain. In the brain, particularly in areas such as the lateral geniculate nucleus (LGN) and the primary visual cortex (V1), these signals are processed further. The LGN acts as a relay station where signals from each eye are combined before being sent to V1 for more complex processing. Here, neurons are organized into orientation-selective and color-selective columns that help in distinguishing between different colors and their orientations. Beyond these physiological mechanisms, biological factors such as age, genetics, and environmental influences can affect color perception. For instance, certain genetic conditions like red-green color blindness result from mutations in genes coding for cone pigments. Additionally, aging can lead to changes in lens transparency and retinal health that alter color perception over time. Cultural and psychological factors also play significant roles in how we perceive colors. Different cultures may associate different meanings with the same color; for example, while white is often associated with purity in Western cultures, it is associated with mourning in many Asian cultures. Psychological studies have shown that emotions and past experiences can influence how we perceive colors as well. In conclusion, the biological perception of color is an intricate process involving both physical structures within the eye and complex neural pathways within the brain. Understanding these mechanisms not only sheds light on how we see but also underscores the dynamic interplay between biology, culture, and psychology that shapes our experience of color in everyday life. This nuanced understanding is crucial for advancing fields such as ophthalmology, neuroscience, and even design where color plays a pivotal role in communication and aesthetics.
3. Chemical Properties of Pigments
Chemical properties of pigments play a crucial role in understanding the concept of color in science. Pigments are substances that change the color of reflected or transmitted light through wavelength-selective absorption. This selective absorption is a result of the pigment's molecular structure, which determines how it interacts with different wavelengths of light. For instance, chlorophyll, a green pigment found in plants, absorbs light in the blue and red parts of the visible spectrum but reflects green light, giving plants their characteristic color. The chemical stability of pigments is another significant property. Some pigments, like titanium dioxide (TiO2), are highly stable and resistant to fading or degradation when exposed to light, water, or other environmental factors. This stability makes them ideal for use in paints and coatings. In contrast, other pigments such as those derived from organic compounds can be more prone to degradation, leading to color changes over time. Understanding these stability issues is essential for applications ranging from art conservation to industrial manufacturing. The reactivity of pigments also influences their color properties. For example, certain pigments can undergo chemical reactions that alter their color when exposed to specific conditions. Prussian blue, a pigment used historically in art, can change its hue if it reacts with acidic or alkaline substances. This reactivity can be both a challenge and an opportunity; while it may lead to unintended color changes, it also allows for the creation of dynamic and responsive materials. Furthermore, the synthesis and purification methods of pigments can significantly impact their chemical properties and hence their color. Modern synthetic methods allow for the production of pigments with precise chemical compositions, leading to consistent and predictable color outcomes. For instance, the development of synthetic iron oxide pigments has enabled the production of a wide range of red, yellow, and brown hues with high purity and uniformity. In addition to these factors, the interaction between pigments and their surroundings can affect their color appearance. Pigments can exhibit different optical effects depending on their particle size and distribution. For example, the phenomenon of scattering, where smaller particles scatter shorter wavelengths more than longer ones, can result in colors that appear more vibrant or iridescent. This is seen in the case of lapis lazuli, a mineral pigment that exhibits a deep blue color due to the scattering of light by its fine particles. Understanding these chemical properties of pigments is fundamental to various scientific disciplines, including materials science, chemistry, and biology. It allows scientists to develop new materials with specific optical properties, enhance the durability of colored products, and even explore the biological functions of pigments in living organisms. By delving into the chemical intricacies of pigments, researchers can unlock new possibilities for color technology and deepen our understanding of the complex science behind color itself.
Applications of Color in Various Scientific Fields
Color, a fundamental aspect of our visual experience, plays a pivotal role in various scientific fields, extending far beyond its aesthetic appeal. In biology and ecology, color is crucial for communication, camouflage, and mating behaviors among organisms. For instance, the vibrant plumage of birds and the intricate patterns on butterflies serve as vital signals in their natural habitats. In chemistry and materials science, color is a key indicator of chemical reactions and material properties. The color changes observed during titrations or the synthesis of new materials are essential for understanding chemical processes. Additionally, in physics and optics, color is a manifestation of light's properties and interactions with matter. The study of color in these fields helps us understand phenomena such as refraction, reflection, and absorption, which are fundamental to our comprehension of the physical world. By exploring these diverse applications, we can gain a deeper appreciation for the multifaceted role of color in scientific inquiry. Let us begin by delving into the fascinating realm of color in biology and ecology, where it shapes the very fabric of life on Earth.
1. Color in Biology and Ecology
Color plays a pivotal role in biology and ecology, serving as a multifaceted tool that influences various aspects of life on Earth. In the natural world, color is not merely a visual attribute but a critical component of survival, communication, and adaptation. For instance, in the realm of animal behavior, coloration can be a powerful signal for attracting mates, warning off predators, or blending into the environment for camouflage. The peacock's vibrant plumage is a classic example of sexual selection, where males display their colorful feathers to attract females and demonstrate genetic fitness. Conversely, the chameleon's ability to change color allows it to hide from predators and sneak up on prey, illustrating the adaptive value of color in predator-prey interactions. In plants, color is equally significant. Flowers use a variety of colors to attract pollinators such as bees, butterflies, and hummingbirds. The specific hues and patterns on flowers are often tailored to the visual capabilities of these pollinators; for example, ultraviolet patterns invisible to humans guide bees to nectar-rich areas. This symbiotic relationship highlights how color facilitates the reproduction of plants by ensuring effective pollination. Moreover, color in biology extends beyond visual communication to include physiological and biochemical processes. Chlorophyll, the green pigment in plants, is essential for photosynthesis—the process by which plants convert sunlight into energy. Other pigments like carotenoids and anthocyanins contribute to the diverse range of colors seen in fruits and vegetables, which can signal ripeness or nutritional value to consumers. In ecological contexts, color can influence community dynamics and ecosystem health. For example, the color of water bodies can indicate their health status; algal blooms often turn water green or red due to high levels of certain pigments, signaling potential environmental issues. Similarly, changes in leaf color during autumn can reflect broader climatic patterns and nutrient cycles within forests. The study of color in biology and ecology also has practical applications. In conservation biology, understanding the role of color in animal behavior can inform strategies for protecting endangered species. For instance, knowing that certain species rely on specific colors for mating or territorial displays can help conservationists design more effective habitat restoration projects. In conclusion, color in biology and ecology is a rich and complex field that underscores the intricate relationships between organisms and their environments. By examining how color functions across different biological systems—from individual behaviors to ecosystem processes—scientists gain deeper insights into the natural world and its many wonders. This understanding not only enhances our appreciation for biodiversity but also provides valuable tools for managing ecosystems sustainably and conserving life on Earth.
2. Color in Chemistry and Materials Science
Color plays a pivotal role in both chemistry and materials science, serving as a critical tool for understanding, analyzing, and developing various substances. In chemistry, color is often used as an indicator of chemical reactions and the presence of specific ions or molecules. For instance, the addition of a few drops of phenolphthalein to an acidic solution results in no color change, but when added to a basic solution, it turns pink, indicating the alkaline nature of the substance. This principle is widely used in titration experiments to determine the endpoint of a reaction. Similarly, the color change observed when potassium permanganate is added to hydrogen peroxide indicates the decomposition of the peroxide, highlighting the oxidative properties of permanganate. In materials science, color is integral to the study and application of pigments, dyes, and other colored materials. The development of new pigments with specific optical properties has significant implications for various industries. For example, the creation of high-performance pigments like YInMn blue (Yttrium Indium Manganese Blue) has opened up new avenues in art conservation and energy-efficient coatings. YInMn blue not only exhibits unique reflectivity properties that can help cool buildings by reflecting infrared radiation but also possesses antimicrobial properties, making it a versatile material for diverse applications. The interaction between light and matter at the molecular level is another area where color is crucial. Quantum dots, tiny semiconductor particles, emit light of specific wavelengths when excited, leading to vibrant colors that can be tuned by adjusting their size. These nanoparticles are being explored for their potential in biomedical imaging, where they can be used to tag cells or tissues with high precision due to their stable and intense fluorescence. Furthermore, the study of color in materials science extends to the realm of structural colors, which arise from the physical structure of materials rather than pigmentation. Butterfly wings and opals are natural examples where microscopic structures create iridescent colors through diffraction and interference of light. Researchers are mimicking these natural phenomena to develop novel materials with dynamic color properties, such as self-healing coatings and adaptive camouflage materials. In addition to these scientific applications, color in chemistry and materials science also has practical implications in everyday life. For instance, the development of color-changing textiles that respond to environmental conditions like temperature or humidity could revolutionize smart clothing technology. Similarly, colorimetric sensors that change color in response to specific analytes are being developed for rapid detection of pollutants or toxins in water and air. In conclusion, the role of color in chemistry and materials science is multifaceted and far-reaching. From serving as indicators in chemical reactions to enabling the development of advanced materials with unique optical properties, color continues to be a vital tool for scientists and engineers. Its applications span from fundamental research to practical innovations that impact various aspects of our lives, underscoring the significance of color as a fundamental aspect of scientific inquiry.
3. Color in Physics and Optics
Color in physics and optics is a multifaceted and intriguing subject that underpins various scientific applications. At its core, color is a manifestation of light's interaction with matter, governed by the principles of electromagnetic radiation. When light, which is part of the electromagnetic spectrum, hits an object, some wavelengths are absorbed while others are reflected or transmitted. This selective interaction determines the color we perceive. In physics, the study of color involves understanding the behavior of light across different wavelengths, typically categorized into the visible spectrum ranging from approximately 380 nanometers (violet) to 780 nanometers (red). One of the fundamental concepts in the physics of color is the theory of additive and subtractive color mixing. Additive mixing, as seen in digital displays like monitors and televisions, combines red, green, and blue (RGB) light to produce a wide range of colors. Conversely, subtractive mixing, used in printing processes, involves combining cyan, magenta, and yellow (CMY) inks to absorb certain wavelengths of light and reflect others, thereby creating the perceived colors. This dichotomy highlights the dual nature of color perception: one based on light emission and the other on pigment absorption. In optics, the study of color extends to phenomena such as dispersion and diffraction. Dispersion occurs when white light passes through a prism or a water droplet, separating into its constituent colors due to differences in refractive indices for different wavelengths. This principle is beautifully illustrated by rainbows, where sunlight is dispersed through water droplets in the air. Diffraction gratings also exploit this property to split light into its spectral components, allowing for precise analysis of light's composition. The applications of color in physics and optics are vast and diverse. Spectroscopy, for instance, relies on the analysis of light spectra to identify chemical compositions and study atomic structures. In astronomy, spectroscopic techniques help scientists determine the chemical makeup of distant stars and galaxies. Additionally, color plays a crucial role in medical imaging technologies like optical coherence tomography (OCT), which uses low-coherence interferometry to create high-resolution images of tissues based on their optical properties. Furthermore, advancements in materials science have led to the development of novel materials with unique optical properties, such as metamaterials and photonic crystals. These materials can manipulate light in ways that natural materials cannot, enabling innovations like ultra-thin optical filters and high-efficiency solar cells. The understanding of color in these contexts not only enhances our technological capabilities but also deepens our understanding of the fundamental laws governing light and matter interactions. In conclusion, the study of color in physics and optics is a rich field that intertwines theoretical principles with practical applications. From the basic mechanisms of light interaction with matter to sophisticated technologies leveraging these principles, color science continues to drive innovation across various scientific disciplines. Its impact is evident in fields ranging from spectroscopy and medical imaging to materials science and astronomy, underscoring the pivotal role that color plays in advancing our understanding of the physical world.
The Role of Color in Scientific Communication and Education
The role of color in scientific communication and education is multifaceted and pivotal, enhancing the clarity, engagement, and retention of scientific information. Color plays a crucial role in various aspects of scientific communication, each contributing to a more effective and engaging dissemination of knowledge. Firstly, **Visual Aids in Scientific Presentations** leverage color to make complex data more accessible and understandable, allowing researchers to convey their findings with greater impact. Secondly, **Color Coding in Data Representation** facilitates the interpretation of large datasets by differentiating between variables and trends, making it easier for audiences to grasp intricate patterns. Lastly, **Engaging Students with Colorful Educational Tools** ensures that learning materials are not only informative but also captivating, thereby fostering a deeper interest in scientific subjects among students. By integrating these elements, color significantly enhances the efficacy of scientific communication. Let us delve into the first of these critical areas: how visual aids in scientific presentations utilize color to elevate the presentation of scientific research.
1. Visual Aids in Scientific Presentations
In the realm of scientific communication and education, visual aids play a pivotal role in enhancing the clarity, engagement, and retention of complex scientific information. When integrated thoughtfully into presentations, these tools can significantly elevate the impact of scientific discourse. Visual aids such as diagrams, charts, graphs, images, and videos serve as powerful communicative devices that help scientists convey intricate data and concepts in a more accessible and compelling manner. One of the primary advantages of visual aids is their ability to simplify complex information. For instance, a well-crafted diagram can illustrate the structure of a molecule or the process of a biochemical reaction more effectively than a lengthy verbal explanation. This visual representation not only aids in immediate comprehension but also facilitates better retention of the information by the audience. Moreover, visual aids can be particularly useful in cross-disciplinary presentations where the audience may not have a uniform background in the subject matter. By using clear and concise visuals, presenters can bridge knowledge gaps and ensure that their message is understood by a diverse audience. Color, in particular, is a critical component of visual aids in scientific presentations. The strategic use of color can highlight key data points, differentiate between various elements, and create visual hierarchies that guide the viewer's attention. For example, in a graph showing experimental results, different colors can be used to represent various variables or data sets, making it easier for the audience to distinguish between them. Additionally, color can be employed to convey emotional or thematic resonance; for instance, using green to represent growth or red to indicate warning or error. Beyond their functional benefits, visual aids also enhance the engagement and interactivity of scientific presentations. Interactive visual tools like 3D models or virtual reality simulations can immerse the audience in the scientific environment, fostering a deeper understanding and interest in the subject matter. These interactive elements can also encourage audience participation and stimulate discussions, turning a passive listening experience into an active learning process. Furthermore, the advent of digital technologies has expanded the repertoire of visual aids available to scientists. Tools such as presentation software, data visualization platforms, and multimedia resources offer a wide range of options for creating dynamic and engaging visuals. These technologies allow presenters to update their visuals in real-time, incorporate live data feeds, and share their presentations across various platforms, thereby extending their reach and impact. In conclusion, visual aids are indispensable in scientific presentations as they facilitate clearer communication, enhance audience engagement, and improve information retention. By leveraging the power of visual elements—especially color—scientists can make their presentations more effective, engaging, and memorable. As science continues to evolve and become increasingly complex, the role of visual aids will only grow more significant, ensuring that scientific knowledge is communicated with clarity and precision to diverse audiences worldwide.
2. Color Coding in Data Representation
Color coding in data representation is a pivotal tool in scientific communication and education, enhancing the clarity, readability, and interpretability of complex data sets. By assigning specific colors to different variables, categories, or trends, researchers can convey intricate information in a visually appealing and easily understandable manner. This technique leverages the human brain's ability to process visual information more efficiently than text or numbers alone, making it an indispensable asset in fields such as biology, physics, and environmental science. In scientific research, color coding helps to highlight patterns and anomalies that might be overlooked in raw data. For instance, in climate studies, temperature maps often use a gradient of colors to depict variations in temperature across different regions. This visual representation allows scientists and non-experts alike to quickly identify areas of extreme heat or cold, facilitating discussions on climate change impacts. Similarly, in medical research, color-coded images of brain scans can differentiate between healthy and diseased tissues, aiding in the diagnosis and treatment of neurological disorders. The strategic use of color also aids in educational settings by making complex concepts more accessible. In textbooks and educational materials, color-coded diagrams and charts can illustrate scientific principles such as the structure of atoms or the process of photosynthesis. This visual reinforcement helps students to better retain information and understand abstract concepts more intuitively. Moreover, interactive tools like color-coded simulations can engage students in hands-on learning experiences, fostering a deeper appreciation for scientific principles. However, the effective use of color coding requires careful consideration to avoid misinterpretation. For example, choosing colors that are distinguishable for individuals with color vision deficiency (CVD) is crucial. Using a palette that includes shades of blue and yellow rather than red and green ensures that the data remains accessible to a broader audience. Additionally, ensuring that the color scheme is consistent across different visual elements helps maintain coherence and prevents confusion. In conclusion, color coding is a powerful tool in scientific communication and education, enabling the clear and engaging presentation of data. By leveraging the cognitive advantages of visual processing, researchers can communicate complex findings more effectively, while educators can enhance student understanding and engagement. As science continues to advance and data sets grow in complexity, the strategic use of color will remain an essential component of scientific communication, bridging the gap between data and comprehension.
3. Engaging Students with Colorful Educational Tools
Engaging students with colorful educational tools is a pivotal strategy in enhancing the learning experience, particularly in scientific education. The incorporation of vibrant colors into educational materials can significantly boost student engagement and comprehension. When learning is presented in a visually appealing manner, it captures students' attention more effectively than monochromatic or text-heavy resources. For instance, colorful diagrams and illustrations in science textbooks help students visualize complex concepts such as cellular structures, molecular interactions, and ecological systems. These visual aids not only make the subject matter more interesting but also facilitate better retention of information. Moreover, interactive tools like colorful flashcards, educational games, and multimedia presentations can transform the classroom into an immersive learning environment. These tools leverage the psychological impact of colors to stimulate curiosity and motivation. For example, using different colors to highlight key terms, processes, or stages in scientific experiments can help students distinguish between various components and understand their interconnections more clearly. Additionally, educational apps and software that utilize color-coded learning modules can provide personalized learning experiences tailored to individual students' needs and learning styles. The use of colorful educational tools also extends beyond traditional classroom settings. Online resources such as educational videos, infographics, and interactive simulations can be designed with color schemes that enhance clarity and engagement. These digital tools are particularly beneficial for remote learning environments where maintaining student interest can be challenging. By incorporating dynamic colors into these resources, educators can create an engaging narrative that keeps students invested in the learning process. Furthermore, the strategic use of colors can cater to diverse learning needs. For students with visual impairments or learning disabilities, high-contrast colors and clear visual distinctions can improve accessibility. Similarly, for students who are visual learners, the judicious use of colors can simplify complex scientific concepts by breaking them down into visually digestible parts. In conclusion, integrating colorful educational tools into scientific education is a powerful approach to fostering student engagement and understanding. By leveraging the cognitive and emotional impact of colors, educators can create a more engaging, accessible, and effective learning environment that prepares students for a deeper appreciation of scientific principles and their applications. This approach not only enriches the educational experience but also lays a strong foundation for lifelong learning in the sciences.