What Does Tdi Stand For
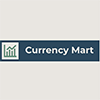
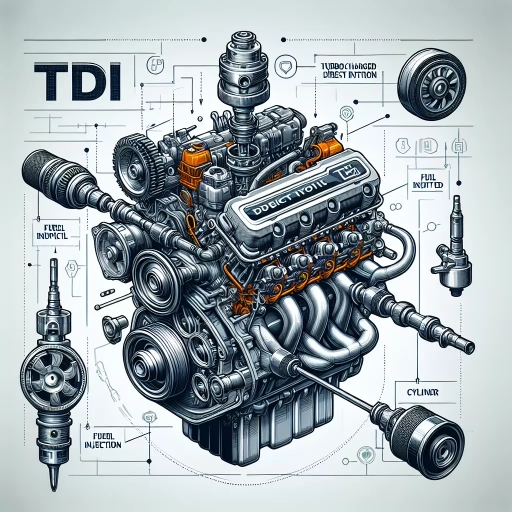
TDI, an acronym that stands for Turbocharged Direct Injection, is a technology that has revolutionized the automotive industry. This innovative approach to engine design combines the benefits of turbocharging and direct fuel injection to enhance performance, efficiency, and environmental sustainability. In this article, we will delve into the multifaceted aspects of TDI, starting with an in-depth explanation of the acronym itself. We will explore the technical applications of TDI, highlighting how it improves engine power and reduces emissions. Additionally, we will examine the real-world implications and impact of TDI technology on both the environment and consumer preferences. By understanding the intricacies of TDI, readers will gain a comprehensive insight into its significance and relevance in modern automotive engineering. Let's begin by **Understanding the Acronym TDI**.
Understanding the Acronym TDI
Understanding the acronym TDI is crucial for grasping its significance in various industrial and environmental contexts. TDI, or Toluene Diisocyanate, is a chemical compound widely used in the production of polyurethane foams, coatings, and elastomers. To fully appreciate its importance, it is essential to delve into its historical context, common uses, and global recognition and standards. Historically, TDI has been a cornerstone in the development of modern materials since its discovery in the early 20th century. Its versatility and unique properties have made it indispensable in industries such as automotive, construction, and consumer goods. Commonly, TDI is utilized in the manufacture of foam cushions, insulation materials, and protective coatings. Additionally, its global recognition is underscored by stringent standards and regulations that govern its use and handling to ensure safety and environmental sustainability. By exploring these facets, we can gain a comprehensive understanding of TDI's role and impact. Let us begin by examining the historical context of TDI, which sets the stage for its widespread adoption and continued relevance today.
Historical Context of TDI
The acronym TDI, standing for Turbocharged Direct Injection, has its roots in the late 20th century when automotive technology was undergoing significant advancements. In the 1980s, Volkswagen Group began developing a new engine technology aimed at improving fuel efficiency and performance. This period was marked by rising fuel prices and increasing environmental concerns, prompting car manufacturers to seek more efficient engine designs. Volkswagen's innovation involved combining turbocharging with direct fuel injection, a concept that had been explored in various forms since the early days of internal combustion engines. However, it was Volkswagen's implementation that made TDI engines commercially viable and widely adopted. The first TDI engine was introduced in 1989 in the Volkswagen Passat, offering a substantial increase in power output while reducing fuel consumption compared to conventional diesel engines. The historical context of TDI is also intertwined with regulatory changes and consumer demand. The 1990s saw stricter emissions regulations being implemented globally, particularly in Europe and North America. TDI technology helped car manufacturers comply with these regulations by reducing emissions while maintaining or improving engine performance. This dual benefit made TDI engines a preferred choice for many consumers seeking both efficiency and power. Throughout the 1990s and early 2000s, Volkswagen continued to refine TDI technology, expanding its application across various models and brands within the Volkswagen Group, including Audi and Skoda. The success of TDI engines contributed significantly to Volkswagen's market share and brand reputation, positioning the company as a leader in diesel engine technology. However, the historical narrative of TDI also includes a notable controversy. In 2015, Volkswagen faced a major scandal known as "Dieselgate," where it was discovered that the company had installed software in its TDI engines to cheat on emissions tests. This led to significant legal and financial repercussions for Volkswagen, as well as a reevaluation of diesel engine technology's environmental impact. Despite this setback, the legacy of TDI continues to influence automotive engineering. The technology has evolved, with ongoing improvements in emissions control and fuel efficiency. Today, TDI remains a relevant term in the automotive industry, symbolizing a blend of performance and efficiency that has shaped the development of modern diesel engines. Understanding the historical context of TDI provides insight into how technological innovation can be driven by both regulatory pressures and consumer needs, highlighting the complex interplay between engineering advancements and societal demands.
Common Uses of TDI
Toluene Diisocyanate (TDI) is a versatile chemical compound with a wide range of applications, primarily due to its reactivity and ability to form strong bonds. One of the most common uses of TDI is in the production of polyurethane foams, which are integral components in various industries. In the automotive sector, TDI-based foams are used for seat cushions, headrests, and other interior components due to their excellent cushioning properties and durability. Similarly, in the construction industry, these foams serve as insulation materials, enhancing energy efficiency and thermal comfort in buildings. In the furniture industry, TDI is crucial for manufacturing flexible foams used in mattresses, sofas, and other upholstered products. Its ability to provide comfort and support makes it an essential material for these applications. Additionally, TDI is used in the production of coatings and adhesives, where its strong bonding capabilities are leveraged to create durable and resistant surfaces. This is particularly beneficial in industrial settings where high-performance coatings are required. TDI also plays a significant role in the textile industry, where it is used to produce spandex fibers. These fibers are known for their elasticity and are commonly found in clothing such as stretch jeans, sportswear, and hosiery. The use of TDI in spandex production ensures that these fabrics retain their shape and provide the necessary flexibility. Furthermore, TDI is utilized in the manufacture of elastomers, which are used in various applications including seals, gaskets, and other rubber products. Its chemical properties make it an ideal choice for creating materials that need to withstand mechanical stress and environmental conditions. In medical applications, TDI-based materials are used in the production of wound dressings and other medical devices due to their biocompatibility and resistance to degradation. This ensures that these medical products can perform their intended functions without causing adverse reactions. Overall, the versatility of TDI makes it a critical component across multiple industries, from automotive and construction to textiles and healthcare. Its unique chemical properties allow it to be tailored for a variety of uses, making it an indispensable material in modern manufacturing processes.
Global Recognition and Standards
Global recognition and standards play a crucial role in the context of understanding the acronym TDI, particularly when it pertains to industries such as automotive, healthcare, or technology. TDI, which stands for Turbocharged Direct Injection, is a technology developed by Volkswagen Group that combines turbocharging and direct fuel injection to enhance engine performance and efficiency. However, the broader significance of global recognition and standards becomes evident when considering the regulatory frameworks and consumer expectations that govern such technologies. In the automotive sector, for instance, global standards ensure that vehicles meet safety, emissions, and performance criteria across different regions. Organizations like the International Organization for Standardization (ISO) and the Society of Automotive Engineers (SAE) establish benchmarks that manufacturers must adhere to. These standards facilitate the seamless integration of technologies like TDI into various markets, ensuring that they comply with local regulations and consumer demands. For example, Euro 6 emissions standards in Europe and EPA standards in the United States dictate the permissible levels of pollutants from vehicle exhausts, influencing how TDI engines are designed and implemented. Similarly, in healthcare, global standards are critical for medical devices and pharmaceuticals. The International Electrotechnical Commission (IEC) and the World Health Organization (WHO) set guidelines that ensure medical products are safe, effective, and consistent worldwide. This uniformity is essential for maintaining public trust and facilitating international trade. When a medical device or drug gains global recognition, it signifies compliance with rigorous standards, which can be a significant factor in its adoption and acceptance. In technology, standards are equally vital. Bodies like the Internet Engineering Task Force (IETF) and the World Wide Web Consortium (W3C) develop protocols that enable interoperability across different systems and platforms. This ensures that technologies can be integrated seamlessly into various environments without compromising functionality or security. For instance, the adoption of HTML5 as a global standard for web development has enabled consistent user experiences across different browsers and devices. The importance of global recognition extends beyond technical compliance; it also impacts consumer perception and market acceptance. Products or technologies that meet international standards are often viewed as more reliable and trustworthy. This can significantly influence purchasing decisions, as consumers tend to prefer products that have been vetted by reputable global authorities. In the case of TDI engines, their compliance with stringent emissions standards has helped in gaining consumer trust and regulatory approval, thereby enhancing their market viability. In summary, global recognition and standards are foundational elements that underpin the development, implementation, and acceptance of technologies like TDI. By adhering to these standards, manufacturers ensure that their products are not only compliant with regulations but also meet the expectations of a diverse global consumer base. This alignment with international benchmarks is crucial for fostering trust, facilitating trade, and driving innovation across various industries.
Technical Applications of TDI
The technical applications of Toluene Diisocyanate (TDI) are multifaceted and critical in various industrial sectors. TDI, a key component in the production of polyurethane foams, coatings, and elastomers, owes its versatility to its unique chemical composition and properties. Understanding the chemical structure and reactivity of TDI is essential for optimizing its use in different applications. Additionally, the industrial manufacturing processes involved in producing TDI are complex and require precise control to ensure consistent quality. Furthermore, environmental and safety considerations are paramount due to the potential health hazards associated with TDI exposure. This article delves into these three crucial aspects, starting with an in-depth examination of the chemical composition and properties of TDI, which form the foundation for its diverse technical applications. By understanding these properties, we can better appreciate how TDI is utilized across various industries and the importance of careful handling and production processes.
Chemical Composition and Properties
TDI, or Toluene Diisocyanate, is a versatile chemical compound with a complex chemical composition and diverse properties that underpin its technical applications. Chemically, TDI is a diisocyanate derived from toluene, characterized by its molecular formula C9H6N2O2. It exists in two main isomers: 2,4-TDI and 2,6-TDI, with the former being more prevalent due to its lower production costs and higher reactivity. The chemical structure of TDI features two isocyanate groups (-NCO) attached to a benzene ring, which are highly reactive and form the basis of its utility in various industrial processes. The properties of TDI are crucial for its technical applications. It is a colorless to pale yellow liquid with a pungent odor, highly reactive towards compounds containing active hydrogen atoms such as alcohols, amines, and water. This reactivity allows TDI to form polyurethane polymers when combined with polyols, making it a key component in the production of foams, coatings, adhesives, and elastomers. The physical properties of TDI include a boiling point around 251°C and a flash point of approximately 127°C, indicating its volatility and flammability. Additionally, TDI has a relatively low viscosity, which facilitates its handling and processing in industrial settings. In terms of safety and environmental considerations, TDI is classified as a hazardous substance due to its potential health risks. Exposure to TDI can cause respiratory problems, skin irritation, and allergic reactions. Therefore, stringent safety protocols are necessary when handling this chemical. Despite these challenges, the unique chemical composition and properties of TDI make it indispensable in various technical applications. For instance, in the automotive industry, TDI-based polyurethane foams are used in seat cushions and dashboards due to their excellent durability and comfort. In construction, TDI-based coatings provide protective barriers against corrosion and wear. Furthermore, in the textile industry, TDI is used to produce elastic fibers and flexible foams for clothing and upholstery. The versatility of TDI also extends to medical applications where its biocompatibility allows it to be used in the production of medical devices such as wound dressings and implantable devices. The ability of TDI to form strong bonds with other molecules makes it an ideal choice for creating durable yet flexible materials that can withstand various environmental conditions. In summary, the chemical composition and properties of TDI are fundamental to its widespread use across multiple industries, highlighting its importance as a critical raw material in modern manufacturing processes.
Industrial Manufacturing Processes
Industrial manufacturing processes are the backbone of modern production, enabling the efficient and precise creation of goods on a large scale. These processes involve a series of complex steps, from raw material selection and processing to final product assembly and quality control. At the heart of many industrial manufacturing processes is the use of technical materials like TDI (Toluene Diisocyanate), a key component in the production of polyurethane foams, elastomers, and coatings. TDI is crucial in various technical applications due to its unique chemical properties. In foam production, TDI reacts with polyols to form polyurethane foams used in insulation, cushioning, and packaging materials. Its ability to create lightweight yet durable foams makes it an essential material in industries such as construction, automotive, and consumer goods. For instance, in the automotive sector, TDI-based foams are used in car seats and dashboards for their excellent shock absorption and comfort. In elastomer production, TDI enhances the flexibility and abrasion resistance of materials, making them ideal for applications like conveyor belts, hoses, and seals. The versatility of TDI also extends to coatings, where it improves the adhesion, hardness, and chemical resistance of paints and varnishes. This makes TDI-coated products highly durable and suitable for use in harsh environments. The integration of TDI into industrial manufacturing processes is facilitated by advanced technologies such as computer-aided design (CAD), 3D printing, and robotic assembly. These technologies allow for precise control over the manufacturing process, ensuring consistent quality and reducing production time. Additionally, automation in manufacturing lines enables the safe handling of TDI, which is a hazardous chemical requiring careful management to prevent exposure and environmental contamination. Quality control is another critical aspect of industrial manufacturing processes involving TDI. Advanced testing methods such as spectroscopy and chromatography are used to ensure that the final products meet stringent standards for performance and safety. This includes testing for mechanical properties, chemical stability, and environmental impact. In conclusion, TDI plays a pivotal role in various industrial manufacturing processes due to its unique properties and versatility. Its applications span multiple industries, from construction to automotive, contributing significantly to the efficiency and quality of modern production. The integration of advanced technologies and rigorous quality control measures further enhances the effectiveness of TDI in these processes, underscoring its importance in the technical applications of industrial manufacturing.
Environmental and Safety Considerations
In the context of Technical Applications of TDI (Toluene Diisocyanate), environmental and safety considerations are paramount. TDI, a key component in the production of polyurethane foams, coatings, and elastomers, poses significant risks if not handled properly. From an environmental perspective, TDI is classified as a hazardous air pollutant by the U.S. Environmental Protection Agency (EPA) due to its potential to cause respiratory problems and other health issues. Emissions from TDI manufacturing and application processes must be strictly controlled to prevent air pollution. Additionally, waste management is critical; any residual TDI or by-products must be disposed of according to stringent regulations to avoid contamination of soil and water. Safety considerations are equally important. TDI is highly reactive and can release harmful vapors, making it essential to use personal protective equipment (PPE) such as respirators, gloves, and protective clothing when handling it. Workers exposed to TDI are at risk of developing occupational asthma and other respiratory diseases if proper ventilation and safety protocols are not followed. The Occupational Safety and Health Administration (OSHA) sets specific standards for permissible exposure limits to TDI in the workplace, emphasizing the need for regular monitoring and enforcement of these limits. Furthermore, the storage and transportation of TDI require careful planning to prevent accidents. Containers must be sealed tightly and labeled correctly, while transportation vehicles should be equipped with appropriate safety measures to handle potential spills or leaks. Emergency response plans must also be in place to address any incidents involving TDI. In terms of technical applications, manufacturers are increasingly adopting safer alternatives and more sustainable practices. For instance, some companies are exploring the use of bio-based raw materials to reduce the environmental footprint of polyurethane production. Others are developing new technologies that minimize TDI emissions during the manufacturing process. Overall, while TDI remains a crucial chemical in various industrial applications, its use must be balanced with rigorous environmental and safety protocols to protect both human health and the environment. By adhering to strict regulations, implementing best practices, and continuously innovating safer technologies, industries can mitigate the risks associated with TDI while leveraging its technical benefits.
Real-World Implications and Impact
The real-world implications and impact of various technologies and innovations are multifaceted, influencing different aspects of society and industry. This article delves into three critical areas: the economic significance in various industries, health and safety regulations, and future trends and innovations. Understanding the economic significance is crucial as it highlights how technological advancements can transform industries such as manufacturing, healthcare, and finance, leading to increased productivity and competitiveness. Health and safety regulations are equally important, as they ensure that new technologies are implemented in a manner that protects workers and consumers from potential risks. Finally, exploring future trends and innovations provides insights into how emerging technologies will shape the landscape of different sectors. By examining these areas, we can better understand the comprehensive impact of technological progress on our economy, well-being, and future development. This article begins by exploring the economic significance in various industries, demonstrating how technological advancements are driving growth and efficiency across diverse sectors.
Economic Significance in Various Industries
Economic significance plays a pivotal role across various industries, influencing not only the financial health of individual sectors but also the broader economy. In the **manufacturing sector**, economic significance is evident through the creation of jobs, stimulation of innovation, and contribution to GDP. For instance, the automotive industry is a significant economic driver in many countries, supporting a vast supply chain and generating substantial revenue. Similarly, in **agriculture**, economic importance is seen in food security, employment opportunities, and export earnings. The agricultural sector often serves as a backbone for rural economies and can significantly impact national food policies. In **technology and IT**, economic significance is reflected in the development of new products and services that drive productivity and efficiency across other industries. Tech giants like Google, Apple, and Microsoft not only generate billions in revenue but also foster innovation ecosystems that spawn startups and create new job markets. The **healthcare industry** is another critical sector where economic significance is multifaceted; it includes the development of life-saving treatments, creation of employment opportunities for medical professionals, and substantial contributions to national healthcare budgets. The **energy sector** holds immense economic significance due to its role in powering other industries and daily life. The extraction, production, and distribution of energy resources such as oil, gas, and renewable energy sources are crucial for economic stability and growth. Moreover, the **financial services sector** acts as a lubricant for the entire economy by facilitating transactions, providing capital for businesses, and managing risk through various financial instruments. In **tourism**, economic significance is seen in the creation of jobs, generation of foreign exchange earnings, and stimulation of local economies. Countries with strong tourism industries often experience significant economic benefits from visitor spending on accommodations, dining, and attractions. Lastly, the **construction industry** is vital for economic growth as it provides infrastructure necessary for other sectors to operate efficiently. It also generates employment opportunities and contributes to urban development. Each of these industries has real-world implications and impacts that extend beyond their immediate economic contributions. They influence consumer behavior, shape policy decisions, and contribute to societal well-being. Understanding the economic significance of these sectors is essential for policymakers, business leaders, and individuals seeking to navigate the complexities of modern economies effectively.
Health and Safety Regulations
Health and Safety Regulations are crucial in ensuring the well-being of employees and the public in various industries. These regulations, often mandated by government agencies such as the Occupational Safety and Health Administration (OSHA) in the United States, set standards for workplace safety, hazardous material handling, and emergency preparedness. For instance, in the construction industry, regulations dictate the use of personal protective equipment (PPE) like hard hats, gloves, and safety glasses to prevent injuries. Similarly, in manufacturing, regulations govern the safe operation of machinery and the proper ventilation of workspaces to mitigate exposure to harmful substances. Compliance with these regulations not only reduces the risk of accidents and illnesses but also enhances productivity and employee morale. Non-compliance can result in severe penalties, legal liabilities, and reputational damage. Furthermore, health and safety regulations have a broader societal impact by contributing to a healthier workforce, reducing healthcare costs, and promoting a culture of safety that extends beyond the workplace. For example, the implementation of ergonomic standards has led to a significant reduction in work-related musculoskeletal disorders, improving overall quality of life for workers. Additionally, regulations on chemical exposure have safeguarded communities from environmental hazards, underscoring the importance of stringent health and safety protocols in protecting both workers and the general public. In summary, health and safety regulations are essential for maintaining a safe and healthy environment, fostering a responsible business culture, and ensuring long-term benefits for individuals and society as a whole.
Future Trends and Innovations
As we delve into the future trends and innovations, it becomes clear that technological advancements will significantly shape various aspects of our lives. One of the most promising areas is artificial intelligence (AI), which is expected to become even more integrated into daily life, enhancing efficiency and decision-making across industries. For instance, AI-driven healthcare will lead to personalized medicine, predictive diagnostics, and automated patient care, revolutionizing the way we approach health and wellness. Another trend on the horizon is the widespread adoption of the Internet of Things (IoT), where interconnected devices will create smart environments that optimize energy consumption, security, and convenience. This will be particularly evident in smart cities, where IoT technologies will manage traffic flow, waste management, and public services more effectively. Sustainable energy solutions are also set to play a crucial role in future innovations. Renewable energy sources such as solar and wind power will continue to gain traction, supported by advancements in battery technology and energy storage systems. Electric vehicles (EVs) will become more mainstream, reducing carbon emissions and transforming the automotive industry. In addition, blockchain technology is poised to disrupt traditional financial systems by offering secure, transparent, and decentralized transactions. This will have profound implications for financial inclusion, supply chain management, and cybersecurity. The rise of augmented reality (AR) and virtual reality (VR) will redefine entertainment, education, and even workplace training. These technologies will provide immersive experiences that enhance learning outcomes and improve job readiness. Furthermore, advancements in biotechnology will lead to breakthroughs in gene editing tools like CRISPR, enabling precise modifications to the human genome and potentially curing genetic diseases. This field also holds promise for regenerative medicine and personalized therapies. Lastly, quantum computing is on the cusp of revolutionizing data processing capabilities, solving complex problems that are currently unsolvable with traditional computers. This will have far-reaching implications for fields such as cryptography, materials science, and climate modeling. These future trends and innovations will have profound real-world implications and impacts. They will drive economic growth, improve quality of life, and address some of the world's most pressing challenges such as climate change and healthcare disparities. As these technologies continue to evolve and mature, it is essential to consider their ethical, social, and environmental impacts to ensure that their benefits are equitably distributed and their risks are mitigated.