What Is Lif
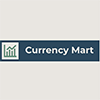
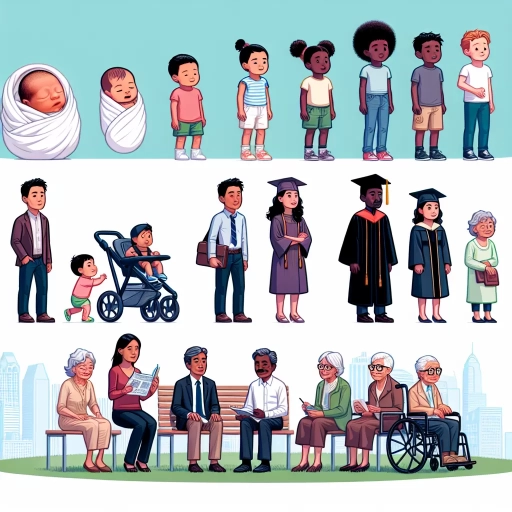
Life, a phenomenon that has captivated human curiosity for centuries, is a multifaceted and intricate concept that encompasses various dimensions. At its core, understanding life involves delving into its fundamental nature, which is explored in the section "Understanding the Concept of Life." This foundational knowledge sets the stage for a deeper examination of the components and processes that sustain life, as discussed in "The Components and Processes of Life." Here, we uncover the essential elements such as cells, metabolism, and reproduction that define living organisms. Furthermore, life's diversity and complexity are highlighted in "The Diversity and Complexity of Life," where we explore the vast array of species, ecosystems, and evolutionary adaptations that make life on Earth so rich and dynamic. By grasping these interconnected aspects, we can gain a comprehensive understanding of what it means to be alive. Let us begin this journey by first exploring the essence of life itself in "Understanding the Concept of Life."
Understanding the Concept of Life
Understanding the concept of life is a multifaceted endeavor that encompasses various disciplines and perspectives. At its core, the definition of life can be approached from biological, philosophical, and scientific angles. Biologically, life is characterized by specific criteria such as organization, metabolism, homeostasis, growth, reproduction, response to stimuli, and evolution. This framework provides a clear and empirical basis for identifying living organisms. Philosophically, the concept of life delves into deeper questions about existence, consciousness, and the human condition, often leading to diverse interpretations and debates. Scientifically, theories on the origin of life seek to explain how the first living cells emerged, offering insights into the primordial conditions and chemical processes that gave rise to life on Earth. By exploring these three dimensions—biological, philosophical, and scientific—we gain a comprehensive understanding of what it means to be alive. Let us begin by examining the biological definition of life, which serves as the foundational framework for our broader exploration.
Biological Definition of Life
**Understanding the Concept of Life** ### Biological Definition of Life The biological definition of life is a multifaceted concept that encompasses several key characteristics and processes. At its core, life is defined by the presence of specific biological attributes that distinguish living organisms from non-living entities. These attributes include **organization**, **metabolism**, **homeostasis**, **growth and development**, **reproduction**, **response to stimuli**, and **evolution**. **Organization** refers to the hierarchical structure of living organisms, ranging from cells to tissues, organs, and systems. Cells, the basic units of life, are organized into complex structures that perform specialized functions. **Metabolism** involves the biochemical processes that sustain life, including anabolism (the synthesis of organic molecules) and catabolism (the breakdown of organic molecules). This metabolic activity is essential for energy production and the synthesis of biomolecules necessary for growth and maintenance. **Homeostasis** is the ability of living organisms to maintain a stable internal environment despite changes in the external environment. This regulatory mechanism ensures that physiological processes operate within optimal ranges, which is crucial for survival. **Growth and development** are characterized by an increase in size and complexity, often involving differentiation into specialized forms or structures. This process is driven by cell division and differentiation. **Reproduction** is a fundamental aspect of life, allowing organisms to produce offspring either sexually or asexually. This ensures the continuation of genetic material from one generation to the next. **Response to stimuli** indicates that living organisms can detect and react to changes in their environment, which aids in survival and adaptation. This responsiveness can range from simple reflexes in single-celled organisms to complex behaviors in multicellular organisms. Finally, **evolution** is the process by which populations of organisms change over time through genetic variation and natural selection. This dynamic process drives the diversity of life on Earth, enabling organisms to adapt to changing environments and leading to the development of new species. Understanding these biological attributes provides a comprehensive framework for defining life. These characteristics are not mutually exclusive; they often interplay and influence one another, creating the intricate web of processes that define living organisms. By recognizing these fundamental principles, we gain insight into what it means to be alive and how life sustains itself across diverse forms and environments. This understanding is crucial not only for appreciating the complexity of life but also for advancing fields such as medicine, ecology, and biotechnology.
Philosophical Perspectives on Life
Philosophical perspectives on life offer a rich tapestry of insights that delve into the very essence and meaning of existence. From ancient Greek philosophers like Aristotle and Plato, who pondered the nature of reality and the human condition, to modern thinkers such as Jean-Paul Sartre and Martin Heidegger, who explored existentialism and the concept of "being," these perspectives provide a multifaceted understanding of life. Aristotle's concept of "eudaimonia" (often translated as happiness or flourishing) suggests that life's purpose is to achieve a state of well-being through virtuous living. In contrast, Plato's theory of forms posits that the material world is merely a shadow of a higher, eternal realm, implying that true life is found in the realm of abstract Forms. Existentialist philosophers like Sartre and Heidegger introduce a more subjective and individualistic view. Sartre's notion of "existence precedes essence" argues that humans are free to choose their actions and create their own meaning in life, without any inherent or predetermined purpose. Heidegger's concept of "Being-in-the-world" emphasizes the fundamental relationship between human existence and the world around us, suggesting that understanding life involves acknowledging our temporal and spatial existence. Eastern philosophies, such as Buddhism and Taoism, offer distinct yet complementary views. Buddhism focuses on the impermanence of all things and the pursuit of enlightenment through mindfulness and detachment from worldly desires. The Taoist concept of "wu wei" (non-action) encourages living in harmony with the natural order of the universe, suggesting that life's meaning is found in aligning oneself with the Tao. The Stoics, with their emphasis on reason and indifference to external events, advocate for a life of inner strength and resilience. They argue that true happiness comes from within and that one should focus on what can be controlled while accepting what cannot with equanimity. In contemporary philosophy, thinkers like Daniel Dennett and Richard Dawkins have contributed to the discussion with a more scientific and evolutionary perspective. Dennett's theory of "memes" as cultural equivalents of genes highlights how ideas and behaviors evolve over time, influencing how we understand human life within a broader evolutionary context. Dawkins' work on evolutionary biology underscores the biological basis of life, emphasizing that our existence is part of an ongoing process shaped by natural selection. These diverse philosophical perspectives collectively enrich our understanding of life by offering various lenses through which to view existence. Whether through the lens of virtue ethics, existential freedom, spiritual enlightenment, or scientific inquiry, each perspective contributes to a deeper comprehension of what it means to be alive. Ultimately, they remind us that the concept of life is complex and multifaceted, inviting continuous reflection and exploration as we seek to understand our place within the grand tapestry of existence.
Scientific Theories of Life's Origin
The scientific theories of life's origin are multifaceted and have evolved significantly over the centuries, offering a rich tapestry of explanations for how life first emerged on Earth. One of the earliest and most influential theories is the **Primordial Soup Hypothesis**, proposed by Alexander Oparin in 1924 and later supported by Stanley Miller and Harold Urey in 1953. This theory suggests that life began in a primordial soup of organic molecules, which were formed through chemical reactions involving water, energy, and simple compounds present on Earth billions of years ago. These molecules eventually assembled into more complex structures, such as amino acids, nucleotides, and lipids, which are the building blocks of life. Another compelling theory is the **RNA World Hypothesis**, which posits that RNA (ribonucleic acid) was the first molecule to store and transmit genetic information. According to this theory, RNA could replicate itself and catalyze chemical reactions, effectively serving as both genetic material and enzyme. This hypothesis is supported by the discovery of ribozymes—RNA molecules that can catalyze specific biochemical reactions—suggesting that RNA could have played a central role in the early stages of life. The **Iron-Sulfur World Theory**, proposed by Günter Wächtershäuser, offers a different perspective by suggesting that life began in an environment rich in iron and sulfur compounds. This theory proposes that simple iron-sulfur clusters could have served as catalysts for the formation of more complex organic molecules, eventually leading to the emergence of cellular life. More recent theories include the **Deep-Hot Biosphere Hypothesis**, which suggests that life originated deep within Earth's crust, where water and minerals are abundant. This theory, proposed by Thomas Gold, posits that microorganisms could have thrived in these environments, eventually giving rise to more complex forms of life. Lastly, the **Panspermia Hypothesis** introduces an extraterrestrial element, suggesting that life on Earth originated from elsewhere in the universe. This theory comes in two forms: directed panspermia, where life is intentionally sent to Earth by advanced civilizations, and undirected panspermia, where life-bearing comets or meteorites collide with our planet, seeding it with microbial life. Each of these theories contributes to our understanding of how life might have originated, highlighting the complexity and diversity of scientific thought on this subject. While no single theory fully explains the origin of life, together they provide a comprehensive framework for understanding the intricate processes that likely led to the emergence of life on Earth. This multifaceted approach underscores the ongoing nature of scientific inquiry into one of humanity's most profound questions: what is life?
The Components and Processes of Life
The intricate tapestry of life is woven from a multitude of complex components and processes, each playing a crucial role in the survival, growth, and reproduction of living organisms. At the heart of this complexity lies the cellular structure and function, which serves as the foundational unit of life. However, cells do not operate in isolation; they are part of a larger network that involves metabolic processes and energy production, ensuring that the cell's needs are met through the conversion of nutrients into energy. Additionally, genetic information and heredity provide the blueprint for cellular operations, dictating traits and characteristics that are passed down through generations. Understanding these interrelated aspects is essential for grasping the full spectrum of life's mechanisms. By delving into the cellular structure and function, we can uncover how cells organize and execute their roles, setting the stage for a deeper exploration of metabolic processes and genetic heredity. This journey begins with an examination of cellular structure and function, the building blocks upon which all other biological processes are constructed.
Cellular Structure and Function
Cellular structure and function are the foundational elements that underpin the intricate mechanisms of life. At the heart of every living organism, cells serve as the basic building blocks, each with a unique set of components and processes that enable them to perform a wide array of functions. The cell membrane, a semi-permeable lipid bilayer, encases the cell and regulates the exchange of materials between the cell and its environment. Inside this membrane lies the cytoplasm, a gel-like substance where metabolic reactions occur, and various organelles are suspended. These organelles include the nucleus, which houses the genetic material in eukaryotic cells; mitochondria, often referred to as the powerhouses of the cell due to their role in energy production through cellular respiration; ribosomes, responsible for protein synthesis; and lysosomes, which contain digestive enzymes that break down cellular waste and foreign substances. Chloroplasts in plant cells are specialized for photosynthesis, converting light energy into chemical energy. The endoplasmic reticulum (ER) and Golgi apparatus work together in protein modification, transport, and secretion. Cytoskeleton structures such as microtubules, microfilaments, and intermediate filaments provide structural support, facilitate cell division, and enable cell movement. The interplay between these cellular components is crucial for maintaining homeostasis and ensuring the cell's survival. For instance, during cellular respiration, glucose is broken down in the mitochondria to produce ATP (adenosine triphosphate), which is then utilized by various cellular processes. Protein synthesis involves transcription of DNA in the nucleus followed by translation on ribosomes scattered throughout the cytoplasm or attached to the ER. This complex network of processes allows cells to grow, reproduce, respond to stimuli, and adapt to changing environments. Moreover, cellular communication plays a vital role in coordinating activities within multicellular organisms. Cells communicate through signaling pathways involving hormones, neurotransmitters, and other signaling molecules that bind to specific receptors on target cells. This communication is essential for regulating growth and development, immune responses, and maintaining tissue integrity. In summary, cellular structure and function are intricately linked to form a dynamic system that supports life at its most fundamental level. Understanding these components and their interactions not only sheds light on how life operates but also underscores the remarkable complexity and resilience inherent in living systems. This foundational knowledge is crucial for advancing fields such as medicine, biotechnology, and environmental science, ultimately contributing to our broader understanding of what life is and how it sustains itself.
Metabolic Processes and Energy Production
Metabolic processes are the intricate series of chemical reactions that occur within living organisms to sustain life, and they are crucial for energy production. These processes can be broadly categorized into two main types: catabolism and anabolism. Catabolism involves the breakdown of complex molecules into simpler ones, releasing energy in the form of ATP (adenosine triphosphate), which is the primary energy currency of the cell. This energy is derived from the food we consume, such as carbohydrates, proteins, and fats. For instance, during cellular respiration, glucose—a simple sugar—is broken down through glycolysis, the citric acid cycle, and oxidative phosphorylation to produce ATP. Conversely, anabolism is the synthesis of complex molecules from simpler ones, requiring energy input often in the form of ATP. This process is essential for growth, repair, and maintenance of cellular structures. The efficiency and regulation of metabolic processes are highly dependent on enzymes—biological catalysts that speed up chemical reactions without being consumed by them. Enzymes ensure that metabolic pathways proceed at optimal rates and under specific conditions, allowing cells to adapt to changing environments. Additionally, hormones and other signaling molecules play critical roles in regulating metabolic activities by influencing enzyme activity and gene expression. Energy production in cells is a highly coordinated process involving multiple cellular compartments. Mitochondria, often referred to as the "powerhouses" of the cell, are central to aerobic respiration where oxygen is used to generate ATP efficiently. In contrast, anaerobic respiration occurs in the absence of oxygen and produces less ATP but is essential for muscle cells during intense physical activity when oxygen supply is limited. The balance between catabolic and anabolic processes is tightly regulated to maintain homeostasis—the stable internal environment necessary for life. This balance is influenced by factors such as nutrient availability, hormonal signals, and environmental conditions. For example, during fasting or starvation, the body shifts towards catabolic pathways to break down stored fats and proteins for energy. Conversely, during periods of nutrient abundance, anabolic pathways are favored to build up reserves and support growth. Understanding metabolic processes and energy production is not only fundamental to appreciating the intricacies of life but also has significant implications for human health. Metabolic disorders such as diabetes and obesity result from dysregulation in these processes, highlighting the importance of maintaining a balanced diet and healthy lifestyle. Furthermore, research into metabolic pathways has led to the development of therapeutic strategies aimed at modulating these processes to treat various diseases. In summary, metabolic processes are essential for converting food into energy and building blocks necessary for cellular functions. The interplay between catabolic and anabolic pathways ensures that cells can adapt to changing conditions while maintaining homeostasis. The intricate regulation of these processes by enzymes, hormones, and other factors underscores the complexity and beauty of life's underlying mechanisms.
Genetic Information and Heredity
Genetic information and heredity are fundamental components of life, governing the transmission of traits from one generation to the next. At the heart of this process lies DNA (deoxyribonucleic acid), a molecule that encodes the genetic instructions used in the development and function of all living organisms. DNA is structured as a double helix, with nucleotide bases—adenine (A), thymine (T), cytosine (C), and guanine (G)—pairing in a specific manner to form genes. These genes are segments of DNA that carry information necessary for the synthesis of proteins, which perform a wide array of biological functions. The process of heredity begins with the replication of DNA, ensuring that each new cell receives an identical set of genetic instructions. During cell division, DNA is replicated through a semi-conservative mechanism where the double helix unwinds, and each strand serves as a template for the synthesis of a new complementary strand. This precise replication is crucial for maintaining genetic continuity across generations. Genetic information is expressed through the central dogma: DNA is transcribed into RNA (ribonucleic acid), which is then translated into proteins. This flow of genetic information from DNA to RNA to proteins is essential for the synthesis of enzymes, hormones, structural proteins, and other molecules vital for cellular function. Mutations in DNA can alter this flow, leading to changes in protein structure and function, which may result in genetic disorders or adaptations that confer selective advantages. Heredity is also influenced by Mendelian genetics, which describes how genes are inherited in a predictable manner. Gregor Mendel's laws of segregation and independent assortment explain how alleles (different forms of a gene) are distributed during gamete formation and how they combine in offspring. These principles help predict the likelihood of certain traits being passed down from parents to offspring. Epigenetics, another layer of genetic regulation, involves chemical modifications to DNA or histone proteins that affect gene expression without altering the DNA sequence itself. These modifications can be influenced by environmental factors and lifestyle choices, providing a mechanism through which environmental exposures can impact heredity. In addition to these molecular and genetic mechanisms, heredity is also shaped by evolutionary processes. Natural selection acts on genetic variation within populations, favoring individuals with traits that enhance survival and reproductive success. Over time, this leads to the accumulation of adaptations that define species and their ability to thrive in various environments. Understanding genetic information and heredity is not only crucial for appreciating the complexity of life but also has significant implications for fields such as medicine, agriculture, and biotechnology. Advances in genetic engineering allow for the manipulation of genes to treat diseases, improve crop yields, and develop novel bioproducts. Furthermore, genetic testing enables the identification of genetic predispositions to diseases, facilitating early intervention and personalized healthcare. In summary, genetic information and heredity form the backbone of life's continuity and diversity. Through DNA replication, gene expression, Mendelian inheritance patterns, epigenetic regulation, and evolutionary processes, organisms transmit and adapt their genetic material across generations. This intricate interplay underscores the dynamic nature of life and highlights the importance of genetics in understanding both the fundamental processes of life and its vast array of manifestations.
The Diversity and Complexity of Life
The diversity and complexity of life on Earth are awe-inspiring, reflecting a rich tapestry of biological, ecological, and evolutionary processes. This intricate web of life is underpinned by several key concepts that help us understand and appreciate its full scope. First, the classification of living organisms provides a systematic framework for categorizing the vast array of species, from bacteria to humans, allowing us to recognize patterns and relationships among different forms of life. Second, ecosystems and biodiversity highlight the interconnectedness of living organisms within their environments, showcasing how diverse species interact and depend on each other for survival. Finally, evolutionary adaptations and survival strategies reveal how organisms have developed unique traits over time to thrive in various habitats. By exploring these interconnected themes, we gain a deeper understanding of the dynamic interplay between life forms and their environments. This journey begins with the fundamental step of categorizing living organisms, which sets the stage for a comprehensive exploration of life's diversity and complexity.
Classification of Living Organisms
The classification of living organisms is a fundamental aspect of understanding the diversity and complexity of life. This process, known as taxonomy, involves grouping organisms based on their shared characteristics and evolutionary relationships. The most widely accepted system of classification is the Linnaean system, developed by Carl Linnaeus in the 18th century. This hierarchical system categorizes organisms into several ranks: Domain, Kingdom, Phylum, Class, Order, Family, Genus, and Species. At the highest level, life is divided into three domains: Archaea, Bacteria, and Eukarya. These domains distinguish between prokaryotic cells (Archaea and Bacteria) and eukaryotic cells (Eukarya), which have a true nucleus and other membrane-bound organelles. Within the Eukarya domain, organisms are further classified into several kingdoms, such as Animalia, Plantae, Fungi, and Protista. Each kingdom represents a distinct group of organisms with unique characteristics; for example, Animalia includes multicellular animals with complex body structures, while Plantae encompasses photosynthetic plants. Below the kingdom level, organisms are grouped into phyla based on their body structure and development. For instance, within the animal kingdom, the phylum Chordata includes animals with a notochord (a precursor to a spinal cord), while the phylum Arthropoda includes insects, crustaceans, and arachnids. Classes further refine these groups; for example, within Chordata, the class Mammalia includes mammals characterized by hair and mammary glands. Orders and families provide even more specific categorization. For example, within Mammalia, the order Carnivora includes meat-eating mammals like cats and dogs, while the family Canidae specifically includes dogs and their relatives. Genera and species are the most specific ranks; a genus groups closely related species, and a species represents a distinct group of interbreeding organisms. For instance, the genus *Canis* includes dogs (*Canis lupus familiaris*), wolves (*Canis lupus*), and jackals (*Canis aureus*). Modern taxonomy also incorporates molecular biology techniques such as DNA sequencing to refine classifications based on genetic relationships. This approach has led to significant revisions in our understanding of evolutionary relationships among different groups of organisms. For example, molecular evidence has confirmed that birds are closely related to theropod dinosaurs and should be classified within the same clade. In summary, the classification of living organisms is a dynamic and evolving field that helps us understand the vast diversity of life on Earth. By organizing organisms into a hierarchical system based on their shared characteristics and genetic relationships, we gain insights into their evolutionary history and the intricate web of life that binds them together. This systematic approach not only aids in scientific research but also underscores the complexity and beauty of life's diversity.
Ecosystems and Biodiversity
Ecosystems and biodiversity are intricately linked, forming the fabric of life on Earth. An ecosystem is a complex network of living organisms (plants, animals, and microbes) and non-living components (such as water, soil, and sunlight) that interact within a specific environment. These interactions create a delicate balance that sustains life, from the simplest microbial communities to the most diverse rainforests. Biodiversity, or the variety of life in the world or in a particular habitat or ecosystem, is crucial for maintaining these balances. It encompasses three main levels: genetic diversity within species, species diversity within ecosystems, and ecosystem diversity across different habitats. The diversity within ecosystems ensures resilience and adaptability. For instance, a forest with many tree species can better withstand diseases and pests compared to a monoculture. This diversity also supports a wide range of ecological services, including air and water purification, soil formation, and climate regulation. Ecosystems with high biodiversity are more efficient at recycling nutrients, decomposing organic matter, and stabilizing the climate. Moreover, they provide essential resources such as food, medicine, and timber. Human activities, however, pose significant threats to both ecosystems and biodiversity. Deforestation, pollution, overfishing, and climate change are among the key drivers of biodiversity loss. The loss of species can have cascading effects on ecosystems, leading to reduced ecosystem function and resilience. For example, the decline of pollinator populations can impact plant reproduction and food production. Conservation efforts are critical to mitigate these impacts. Protected areas like national parks and wildlife reserves help preserve biodiversity hotspots. Sustainable practices such as agroforestry and eco-friendly agriculture can also support ecosystem health while meeting human needs. Understanding the intricate relationships within ecosystems and the importance of biodiversity is essential for managing natural resources sustainably. This knowledge informs policies and practices that aim to balance human development with environmental conservation. By recognizing the value of ecosystems and biodiversity, we can work towards preserving the rich tapestry of life that supports our planet's ecological integrity and ensures a healthy future for all living beings. In summary, ecosystems and biodiversity are fundamental to the diversity and complexity of life on Earth. The interconnectedness of living and non-living components in ecosystems creates resilient systems that provide essential services. However, these systems are under threat from human activities. Efforts to conserve and sustainably manage ecosystems are vital for maintaining biodiversity and ensuring the long-term health of our planet. By appreciating and protecting this diversity, we safeguard not only the natural world but also our own well-being and future.
Evolutionary Adaptations and Survival Strategies
Evolutionary adaptations and survival strategies are the cornerstone of life's diversity and complexity, enabling organisms to thrive in a wide range of environments. These adaptations are the result of natural selection, where favorable traits that enhance survival and reproductive success become more common in a population over time. For instance, the peppered moth in England underwent a dramatic shift from light to dark coloration in response to the industrial revolution, which darkened tree bark with soot. This change allowed the dark-colored moths to blend in with their new environment, significantly increasing their chances of survival. In aquatic ecosystems, fish have developed remarkable adaptations such as gills for oxygen extraction from water and streamlined bodies for efficient swimming. Desert-dwelling organisms like cacti have evolved thick, waxy stems to store water and spines to reduce water loss through transpiration. In arctic regions, polar bears possess white fur and a layer of blubber to insulate themselves against extreme cold, while their large paws act as snowshoes to distribute their weight evenly on snow. Survival strategies also include behavioral adaptations. Many species exhibit migratory behaviors to avoid harsh weather conditions or find food sources. For example, monarch butterflies migrate thousands of miles each year from Canada and the United States to Mexico, guided by an innate genetic program. Social behaviors are another critical survival strategy; ants and bees live in highly organized colonies where division of labor ensures the colony's overall survival. Chemical defenses are another form of adaptation. Certain plants produce toxic compounds to deter herbivores, while some animals can synthesize these toxins themselves or acquire them from their diet. The monarch butterfly, for instance, sequesters toxic chemicals from the milkweed plants it consumes as a caterpillar, making it unpalatable to predators as an adult. Furthermore, evolutionary adaptations extend to the molecular level. Enzymes in organisms living in extreme environments, such as deep-sea vents or hot springs, have evolved to function optimally under conditions that would be lethal to most other life forms. These enzymes are often highly stable and efficient at high temperatures or under high pressure. The diversity of life is also reflected in the variety of reproductive strategies that have evolved. Some species, like aphids, can reproduce parthenogenically without males, ensuring rapid population growth in favorable conditions. Others, such as certain species of frogs and toads, exhibit complex mating rituals that involve vocalizations and visual displays to attract mates. In conclusion, evolutionary adaptations and survival strategies are fundamental to understanding the diversity and complexity of life on Earth. These adaptations span from physical traits like camouflage and insulation to behavioral strategies such as migration and social organization, and even molecular adaptations that allow organisms to thrive in extreme environments. Each adaptation represents a unique solution to the challenges posed by an organism's environment, highlighting the incredible versatility and resilience of life.