What Is Biogel
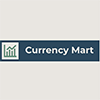
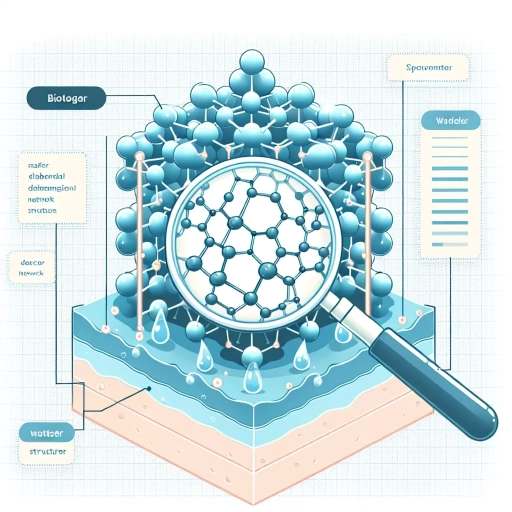
In the realm of advanced materials, biogel stands out as a versatile and innovative substance that has garnered significant attention across various scientific and industrial fields. This high-performance material combines the benefits of both biological and synthetic components, offering unique properties that make it highly valuable in diverse applications. To fully understand the potential of biogel, it is essential to delve into its fundamental aspects. This article will explore what biogel is, starting with an **Introduction to Biogel**, where we will define its core characteristics and historical development. We will then examine the **Composition and Properties of Biogel**, highlighting its molecular structure and the distinct features that set it apart from other materials. Finally, we will discuss the **Uses and Benefits of Biogel**, outlining its applications in fields such as medicine, agriculture, and environmental science. By understanding these key aspects, readers will gain a comprehensive insight into the world of biogel and its transformative impact. Let us begin by diving into the **Introduction to Biogel**.
Introduction to Biogel
In the realm of biomaterials, few innovations have garnered as much attention and potential as biogels. These versatile, gel-like substances are engineered to mimic the properties of natural tissues, offering a wide range of applications in medicine, biotechnology, and beyond. To fully appreciate the significance of biogels, it is essential to delve into their **Definition and Origin**, understanding the fundamental principles that define them and the scientific breakthroughs that led to their discovery. Additionally, exploring the **Historical Development** of biogels provides insight into how these materials have evolved over time, from early experiments to current sophisticated formulations. Finally, examining their **Current Applications** reveals the diverse ways in which biogels are being utilized today, from drug delivery systems and tissue engineering to diagnostic tools and regenerative medicine. This article aims to introduce readers to the fascinating world of biogels, guiding them through these key aspects and illuminating the future possibilities that these biomaterials hold. Welcome to our **Introduction to Biogel**.
Definition and Origin
**Definition and Origin** Biogel, a term that has garnered significant attention in recent years, refers to a type of hydrogel derived from biological sources. These gels are composed of water-absorbing polymers that are either naturally occurring or synthesized from biomolecules such as proteins, polysaccharides, and nucleic acids. The unique properties of biogels, including their biocompatibility, biodegradability, and ability to mimic the extracellular matrix of tissues, make them highly versatile in various biomedical applications. The origin of biogels can be traced back to the early 20th century when scientists began exploring the potential of natural polymers for medical use. However, it was not until the advent of advanced biotechnology and nanotechnology in the late 20th and early 21st centuries that the field of biogel research truly flourished. Researchers discovered that by modifying natural polymers or combining them with synthetic components, they could create gels with tailored mechanical, chemical, and biological properties. This innovation opened up new avenues for tissue engineering, drug delivery systems, wound healing, and even cosmetic applications. One of the key milestones in the development of biogels was the discovery of how to cross-link natural polymers to form stable three-dimensional networks. This process allowed for the creation of gels that could maintain their structure while still being permeable to nutrients and waste products, making them ideal for cell encapsulation and tissue regeneration. For instance, alginate, a polysaccharide derived from seaweed, has been widely used to create biogels due to its ease of cross-linking and excellent biocompatibility. The interdisciplinary nature of biogel research has also contributed to its rapid advancement. Collaboration between bioengineers, chemists, biologists, and clinicians has led to the development of sophisticated biogel systems that can respond to environmental stimuli, release therapeutic agents in a controlled manner, and even promote cellular differentiation. As research continues to evolve, biogels are poised to play an increasingly important role in addressing some of the most pressing challenges in healthcare and biotechnology. In summary, biogels represent a cutting-edge field at the intersection of biology and materials science. Their definition encompasses a broad range of hydrogels derived from biological sources, while their origin is rooted in decades of scientific innovation aimed at harnessing the potential of natural polymers for medical applications. As ongoing research continues to push the boundaries of what is possible with biogels, their impact on healthcare and beyond is likely to be profound.
Historical Development
The historical development of biogels is a fascinating narrative that intertwines advancements in materials science, biotechnology, and medical research. The journey began in the early 20th century with the discovery of hydrogels, which are networks of hydrophilic polymer chains that can absorb and retain large amounts of water. These early hydrogels were primarily used in industrial applications but laid the groundwork for more sophisticated biomaterials. In the 1960s and 1970s, researchers started exploring the potential of hydrogels in biomedical fields, particularly in drug delivery systems and tissue engineering. This period saw significant breakthroughs with the development of synthetic hydrogels such as poly(2-hydroxyethyl methacrylate) (HEMA), which demonstrated biocompatibility and could be tailored to mimic natural tissues. The 1980s marked a pivotal shift with the introduction of natural polymer-based biogels derived from sources like collagen, alginate, and chitosan. These biogels offered enhanced biocompatibility and bioactivity, making them ideal for applications such as wound healing, cell encapsulation, and regenerative medicine. The advent of nanotechnology in the 1990s further refined biogel design, enabling the creation of nanostructured biogels that could interact at the cellular level. This era also witnessed significant advancements in cross-linking techniques, allowing for greater control over biogel properties such as mechanical strength, degradation rate, and drug release profiles. In the 21st century, the field has continued to evolve with the integration of cutting-edge technologies like 3D printing and microfluidics. These tools have enabled the fabrication of complex biogel structures with precise geometries and functionalities, opening new avenues for personalized medicine and tissue engineering. Additionally, the rise of bioactive biogels has introduced materials that can actively interact with biological systems, promoting cellular differentiation, proliferation, and tissue regeneration. Today, biogels are being explored for a wide range of applications including cancer therapy, cardiovascular disease treatment, and even neural tissue repair. The ongoing research in this field promises to revolutionize healthcare by providing innovative solutions that are both effective and biocompatible. As we delve into the world of biogels, it becomes clear that their historical development is a testament to human ingenuity and the relentless pursuit of improving human health through science and technology.
Current Applications
In the realm of biotechnology and biomedical engineering, biogels have emerged as versatile and dynamic materials with a myriad of current applications. These hydrogel-based biomaterials, composed of biopolymers or synthetic polymers, are engineered to mimic the extracellular matrix of tissues, providing a scaffold for cell growth, differentiation, and tissue regeneration. One of the most significant applications of biogels is in tissue engineering and regenerative medicine. Here, they serve as three-dimensional scaffolds that support the growth of cells and tissues, facilitating the repair or replacement of damaged tissues. For instance, biogels are being used in the development of artificial skin for burn victims, as well as in the creation of bioengineered heart valves and vascular grafts. Biogels also play a crucial role in drug delivery systems. Their ability to encapsulate drugs and release them in a controlled manner makes them ideal for targeted therapy. This controlled release mechanism can enhance the efficacy of drugs while minimizing side effects, making biogels particularly useful for treating chronic diseases such as cancer and diabetes. Additionally, biogels are being explored for their potential in biosensors and diagnostic tools. Their responsive nature allows them to change properties in response to environmental stimuli, such as pH or temperature changes, which can be leveraged to detect biomarkers for various diseases. In the field of wound healing, biogels have shown promising results by providing a moist environment that promotes faster healing and reduces the risk of infection. They can also be loaded with growth factors and antimicrobial agents to further enhance the healing process. Furthermore, biogels are being used in cosmetic applications, such as in skincare products where they help retain moisture and improve skin elasticity. The use of biogels extends into the realm of 3D printing and biofabrication as well. Here, they are used as bioinks that can be printed into complex structures mimicking natural tissues. This technology holds great promise for creating personalized organ models for surgical planning and training, as well as for developing functional organs for transplantation. In summary, biogels are multifunctional biomaterials that have revolutionized various fields from tissue engineering and drug delivery to wound healing and cosmetic applications. Their unique properties make them an indispensable tool in modern biomedical research and clinical practice, offering innovative solutions to some of the most pressing health challenges of our time. As research continues to advance, the potential applications of biogels are expected to expand even further, contributing significantly to the development of novel therapeutic strategies and diagnostic tools.
Composition and Properties of Biogel
Biogels, a class of biomaterials that combine the advantages of hydrogels with the functionality of biological molecules, have emerged as a pivotal component in various biomedical applications. These versatile materials are engineered to mimic the extracellular matrix, providing a supportive environment for cell growth and tissue regeneration. The composition and properties of biogels are crucial for their effectiveness, making them a subject of intense research. This article delves into the key aspects of biogels, starting with the **Materials Used in Biogel Formation**, which highlights the diverse range of natural and synthetic polymers employed in their creation. It then explores their **Physical and Chemical Properties**, including their mechanical strength, swelling behavior, and degradation profiles. Finally, it addresses **Biocompatibility and Safety**, a critical factor in ensuring these materials are suitable for use in human tissues. By understanding these facets, researchers and clinicians can better harness the potential of biogels in therapeutic and diagnostic applications. This comprehensive overview sets the stage for a deeper exploration into the world of biogels, introducing readers to the multifaceted nature of these innovative biomaterials. **Introduction to Biogel**.
Materials Used in Biogel Formation
In the formation of biogels, a diverse array of materials is employed to achieve the desired properties and functionalities. These materials can be broadly categorized into natural and synthetic components, each contributing unique characteristics to the biogel matrix. Natural polymers such as collagen, alginate, chitosan, and hyaluronic acid are commonly used due to their biocompatibility, biodegradability, and ability to mimic the extracellular matrix (ECM) of tissues. Collagen, for instance, is a primary structural protein found in connective tissue and provides excellent mechanical strength and cell adhesion sites. Alginate, derived from seaweed, forms hydrogels through ionic cross-linking and is widely used in drug delivery and tissue engineering applications. Chitosan, a polysaccharide obtained from chitin, offers antimicrobial properties and can be cross-linked to form stable hydrogels. Hyaluronic acid, a glycosaminoglycan, is known for its high water-binding capacity and is often used in wound healing and cosmetic products. Synthetic polymers like polyethylene glycol (PEG), poly(lactic-co-glycolic acid) (PLGA), and polyacrylamide are also integral in biogel formation. These materials provide tunable mechanical properties, controlled degradation rates, and can be easily functionalized with bioactive molecules. PEG is particularly useful for its hydrophilicity and ability to form hydrogels through various cross-linking mechanisms, making it suitable for drug delivery systems. PLGA, a copolymer of lactic acid and glycolic acid, is biodegradable and has been extensively used in biomedical applications due to its FDA approval and versatility in formulation. In addition to these polymers, other components such as cells, growth factors, and bioactive molecules are incorporated into biogels to enhance their biological performance. Cells like stem cells or primary cells can be encapsulated within the biogel matrix to promote tissue regeneration or repair. Growth factors such as vascular endothelial growth factor (VEGF) and platelet-derived growth factor (PDGF) are added to stimulate cellular proliferation and differentiation. Bioactive molecules like peptides or small molecules can be integrated to provide specific biological cues that guide cellular behavior. The choice of materials in biogel formation is critical as it directly influences the composition and properties of the resulting biogel. By carefully selecting and combining these materials, researchers can tailor the mechanical strength, degradation rate, cell adhesion properties, and bioactivity of biogels to suit various biomedical applications. This versatility makes biogels highly promising for use in tissue engineering, drug delivery, wound healing, and regenerative medicine, among other fields. Understanding the materials used in biogel formation is essential for optimizing their performance and ensuring their safety and efficacy in clinical settings.
Physical and Chemical Properties
Physical and chemical properties are fundamental aspects that define the composition and behavior of biogels, which are hydrogel-based biomaterials used in various biomedical applications. **Physical Properties** of biogels include their mechanical strength, swelling capacity, and porosity. The mechanical strength is crucial as it determines the durability and stability of the biogel in different environments. For instance, biogels used in tissue engineering need to possess sufficient mechanical integrity to support cell growth and tissue regeneration. Swelling capacity is another key physical property; it influences how the biogel interacts with its surroundings, absorbing fluids and nutrients while releasing therapeutic agents. Porosity affects the diffusion rates of molecules through the biogel matrix, impacting its ability to facilitate cellular migration and nutrient exchange. **Chemical Properties** are equally important and include the chemical composition, cross-linking density, and surface chemistry of the biogel. The chemical composition dictates the biocompatibility and biodegradability of the biogel. For example, biogels made from natural polymers like collagen or alginate are generally more biocompatible compared to those derived from synthetic polymers. Cross-linking density affects the network structure of the biogel, influencing its mechanical properties and degradation rate. Surface chemistry plays a significant role in cell adhesion and proliferation; functional groups on the surface can be modified to enhance or inhibit cellular interactions. The interplay between these physical and chemical properties is critical for tailoring biogels to specific applications. In drug delivery systems, for instance, the chemical composition and cross-linking density can be adjusted to control the release kinetics of therapeutic agents. In wound healing, biogels with high swelling capacity and appropriate surface chemistry can create a moist environment conducive to tissue repair while preventing infection. Understanding these properties allows researchers to design biogels that mimic the extracellular matrix, providing a supportive environment for cellular activities and tissue regeneration. Moreover, the versatility of biogels lies in their ability to be engineered with specific physical and chemical attributes. Advanced techniques such as photo-crosslinking or 3D printing enable precise control over the microstructure and macrostructure of biogels, allowing for customized mechanical properties and spatially controlled release profiles. This adaptability makes biogels highly promising materials for a wide range of biomedical applications, from regenerative medicine to biosensors. In summary, the physical and chemical properties of biogels are pivotal in determining their performance and suitability for various biomedical uses. By carefully engineering these properties, researchers can create biogels that not only mimic natural tissues but also offer enhanced functionalities, making them invaluable tools in advancing healthcare technologies.
Biocompatibility and Safety
**Biocompatibility and Safety** Biocompatibility and safety are paramount considerations when evaluating the suitability of biogels for various applications, particularly in medical and biological contexts. Biogels, being hydrogel-based materials, must interact harmoniously with biological systems to ensure they do not elicit adverse reactions or compromise the health of individuals. The biocompatibility of biogels is assessed through rigorous testing protocols that evaluate their cytotoxicity, genotoxicity, and potential to induce immune responses. One critical aspect of biogel biocompatibility is the selection of its constituent materials. The polymers and cross-linking agents used in biogel synthesis must be non-toxic and free from leachable impurities that could leach into biological fluids. For instance, biogels derived from natural polymers such as alginate, collagen, or hyaluronic acid are often preferred due to their inherent biocompatibility and biodegradability. These materials mimic the extracellular matrix of tissues, promoting cell adhesion and proliferation without causing significant inflammatory responses. In addition to material selection, the physical and chemical properties of biogels play a crucial role in determining their safety profile. The porosity, swelling ratio, and mechanical strength of biogels can influence their interaction with cells and tissues. For example, a biogel with optimal porosity can facilitate nutrient diffusion and waste removal, thereby supporting cellular viability and function. Conversely, a biogel with inappropriate mechanical properties may cause tissue damage or induce fibrotic responses. Regulatory compliance is another essential factor in ensuring the safety of biogels. Manufacturers must adhere to stringent guidelines set by regulatory bodies such as the U.S. Food and Drug Administration (FDA) or the European Medicines Agency (EMA). These guidelines mandate thorough preclinical and clinical testing to validate the biocompatibility and safety of biogels before they are approved for clinical use. Moreover, the sterilization process of biogels is critical to prevent contamination and ensure patient safety. Common sterilization methods include gamma irradiation, ethylene oxide treatment, or autoclaving, each with its own set of advantages and limitations that must be carefully considered based on the specific composition and intended application of the biogel. In summary, the biocompatibility and safety of biogels are multifaceted issues that require meticulous attention to material selection, physical and chemical properties, regulatory compliance, and sterilization protocols. By addressing these factors comprehensively, researchers and manufacturers can develop biogels that are not only effective but also safe for use in a wide range of biomedical applications. This ensures that biogels can fulfill their potential in enhancing human health without posing undue risks to patients.
Uses and Benefits of Biogel
Biogel, a versatile and innovative material, has garnered significant attention across various fields due to its unique properties and wide-ranging applications. This article delves into the multifaceted uses and benefits of biogel, highlighting its impact in medical, environmental, and industrial contexts. In the realm of medical applications, biogel is revolutionizing healthcare by providing advanced solutions for wound healing, drug delivery, and tissue engineering. Its biocompatibility and ability to mimic natural tissues make it an ideal material for medical research and treatment. Beyond healthcare, biogel also plays a crucial role in environmental conservation by aiding in pollution control and sustainable resource management. Its absorbent properties make it effective in cleaning up oil spills and managing water quality. Additionally, biogel's potential in industrial and research applications is vast, with uses in biotechnology, agriculture, and materials science. This article will explore these diverse applications in depth, providing a comprehensive understanding of the uses and benefits of biogel. To fully appreciate the significance of this material, let us begin with an **Introduction to Biogel**.
Medical Applications
Biogel, a versatile and innovative biomaterial, has revolutionized various medical applications due to its unique properties and biocompatibility. One of the most significant uses of biogel is in wound healing. Biogel-based dressings provide a moist environment that promotes faster healing, reduces scarring, and minimizes the risk of infection. These dressings can be tailored to release growth factors and other therapeutic agents directly to the wound site, enhancing tissue regeneration and repair. Additionally, biogel's ability to absorb and retain fluids makes it an ideal component for hydrocolloid dressings, which are particularly effective for chronic wounds such as ulcers and burns. In the field of tissue engineering, biogel serves as a scaffold for cell growth and differentiation. Its porous structure allows cells to adhere, proliferate, and form tissue-like structures, making it a crucial tool for regenerative medicine. Biogel scaffolds can be engineered to mimic the extracellular matrix of specific tissues, providing a supportive environment for the growth of new tissue. This application holds great promise for repairing damaged tissues in conditions such as heart disease, bone fractures, and neurological disorders. Biogel also plays a critical role in drug delivery systems. Its hydrophilic nature allows it to encapsulate a wide range of therapeutic agents, including proteins, peptides, and small molecules. By controlling the release rate of these drugs, biogel-based delivery systems can provide sustained therapeutic effects over extended periods. This is particularly beneficial for chronic conditions where consistent drug levels are essential for effective management. Furthermore, biogel's biodegradability ensures that the delivery system is safely absorbed by the body without causing adverse reactions. In ophthalmology, biogel is used in contact lenses and intraocular lenses due to its excellent optical clarity and biocompatibility. Biogel contact lenses offer superior comfort and vision correction while minimizing the risk of eye irritation. Intraocular lenses made from biogel are used in cataract surgery to replace the natural lens, providing clear vision post-surgery with minimal complications. Moreover, biogel finds applications in dental care. It is used in dental impression materials due to its ability to accurately capture the details of teeth and gums. This ensures precise fitting of dental restorations such as crowns and bridges. Additionally, biogel-based products are used in periodontal treatments to deliver antibiotics directly to the site of infection, enhancing the efficacy of treatment. The benefits of using biogel in medical applications are multifaceted. Its biocompatibility reduces the risk of adverse reactions, making it safer for patients. The ability to tailor biogel properties for specific applications allows for personalized treatment options. Moreover, biogel's role in promoting tissue regeneration and drug delivery enhances treatment outcomes and improves patient quality of life. Overall, the versatility and effectiveness of biogel make it an indispensable material in modern medical practices.
Environmental Uses
Biogel, a versatile and eco-friendly material, offers a myriad of environmental uses that underscore its potential as a sustainable solution for various ecological challenges. One of the most significant environmental applications of biogel is in soil conservation and agriculture. By incorporating biogel into soil, farmers can enhance water retention, reduce soil erosion, and improve soil structure. This not only conserves water but also minimizes the need for frequent irrigation, thereby saving resources and reducing the environmental impact of agricultural practices. Additionally, biogel can act as a carrier for fertilizers and pesticides, allowing for controlled release and minimizing runoff into waterways, which helps in maintaining soil health and preventing water pollution. Another critical environmental use of biogel is in wastewater treatment. Biogel can be engineered to absorb and remove contaminants such as heavy metals, organic pollutants, and other hazardous substances from wastewater. This capability makes it an invaluable tool in cleaning up industrial effluents and ensuring that water discharged into natural ecosystems is safe and free from harmful chemicals. Furthermore, biogel's biodegradable nature means that it does not contribute to long-term environmental degradation, unlike traditional synthetic materials used in water treatment processes. Biogel also plays a crucial role in reforestation efforts and habitat restoration. When used as a seed coating or mixed with soil for planting, biogel helps seeds retain moisture, ensuring higher germination rates and healthier seedlings. This is particularly beneficial in arid or drought-prone areas where water scarcity is a significant challenge. Moreover, biogel can be used to create artificial wetlands or restore degraded habitats by providing a stable medium for plant growth and supporting biodiversity. In the context of climate change mitigation, biogel can be utilized to enhance carbon sequestration. By improving soil health and promoting plant growth, biogel indirectly supports the absorption of carbon dioxide from the atmosphere through photosynthesis. Additionally, biogel-based products can replace traditional materials in packaging and other applications, reducing the reliance on non-renewable resources and lowering greenhouse gas emissions associated with their production. Overall, the environmental uses of biogel are multifaceted and far-reaching. From enhancing agricultural sustainability to cleaning wastewater and supporting reforestation, biogel offers a range of benefits that align with global efforts to protect and preserve our environment. Its biodegradable and non-toxic properties make it an attractive alternative to conventional materials, positioning biogel as a key component in the transition towards more sustainable practices.
Industrial and Research Applications
Biogel, a versatile and innovative material, has found extensive applications in both industrial and research settings due to its unique properties and benefits. In industrial contexts, biogel is utilized for its superior biocompatibility, mechanical strength, and ability to mimic natural tissues. For instance, in the biomedical sector, biogel is used in the development of tissue engineering scaffolds that support cell growth and differentiation, facilitating the creation of artificial tissues for transplantation. This application is particularly significant in regenerative medicine, where biogel-based scaffolds can help repair damaged tissues or organs by providing a supportive matrix for cellular regeneration. In pharmaceuticals, biogel serves as an effective drug delivery system. Its porous structure allows for controlled release of therapeutic agents, ensuring sustained drug delivery over a prolonged period. This enhances the efficacy of treatments while minimizing side effects associated with traditional drug administration methods. Additionally, biogel's biodegradability makes it an attractive material for developing environmentally friendly packaging solutions and disposable products. From a research perspective, biogel offers a robust platform for various scientific investigations. In materials science, researchers study the mechanical properties and degradation kinetics of biogel to optimize its performance in different applications. In biology and biochemistry, biogel is used to create three-dimensional cell culture models that more accurately simulate in vivo conditions compared to traditional two-dimensional cultures. This has revolutionized our understanding of cellular behavior and interactions, enabling more accurate modeling of disease mechanisms and testing of therapeutic interventions. Moreover, biogel's hydrophilic nature makes it an ideal material for biosensors and diagnostic tools. By incorporating specific biomolecules or nanoparticles into the biogel matrix, scientists can develop highly sensitive biosensors capable of detecting biomarkers for diseases such as cancer or infectious diseases. These biosensors offer rapid and accurate diagnostic capabilities, which are crucial for early disease detection and personalized medicine. The versatility of biogel also extends to environmental science, where it is used in wastewater treatment processes. Biogel-based systems can effectively remove contaminants from water by adsorption or enzymatic degradation, contributing to cleaner water resources and a healthier environment. Overall, the multifaceted applications of biogel underscore its potential as a transformative material across diverse fields, from healthcare and pharmaceuticals to research and environmental conservation. Its unique combination of biocompatibility, mechanical strength, and tunable properties makes it an indispensable tool in advancing various technologies and scientific endeavors.