What Does Naturally Aspirated Mean
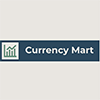
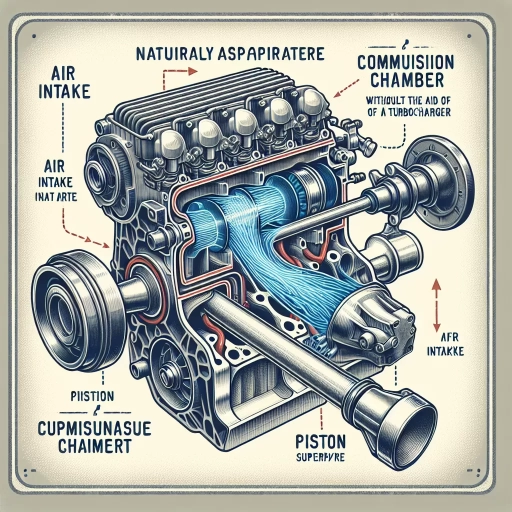
In the world of automotive engineering, the term "naturally aspirated" refers to an engine that relies solely on atmospheric pressure to draw air into the combustion chamber, without the aid of forced induction such as turbochargers or superchargers. This fundamental design principle has significant implications for engine performance, efficiency, and overall application. To delve into the intricacies of naturally aspirated engines, it is essential to understand their underlying mechanics, key components, and how these factors influence their performance characteristics. This article will explore these aspects in depth, starting with a comprehensive overview of **Understanding Naturally Aspirated Engines**, followed by an examination of **Key Components and Mechanics**, and concluding with an analysis of **Performance Characteristics and Applications**. By grasping these core concepts, readers will gain a thorough understanding of what it means for an engine to be naturally aspirated and how this affects its operation and use in various contexts. Let's begin by **Understanding Naturally Aspirated Engines**.
Understanding Naturally Aspirated Engines
Understanding naturally aspirated engines is a multifaceted topic that delves into the heart of automotive engineering. At its core, a naturally aspirated engine relies on atmospheric pressure to draw air into the combustion chamber, contrasting with forced induction methods that use turbochargers or superchargers to compress air. This distinction is crucial when comparing naturally aspirated engines with their forced induction counterparts, as each design has its own set of advantages and limitations. Historically, naturally aspirated engines have been the foundation of automotive powerplants, evolving over decades to achieve higher efficiency and performance. To grasp the essence of these engines, it is essential to start with their definition and basic principles. By understanding how naturally aspirated engines operate at a fundamental level, we can better appreciate their role in the broader context of automotive technology and their ongoing relevance in modern vehicles. Therefore, let us begin by exploring the definition and basic principles of naturally aspirated engines.
Definition and Basic Principles
**Definition and Basic Principles** Understanding naturally aspirated engines begins with a clear grasp of their fundamental definition and operating principles. A naturally aspirated engine, often abbreviated as NA, is a type of internal combustion engine that relies solely on atmospheric pressure to draw air into the combustion chamber. Unlike forced induction engines, which use turbochargers or superchargers to compress and force more air into the engine, naturally aspirated engines depend on the natural flow of air through the intake system. This air is drawn in by the vacuum created by the pistons moving downward in the cylinders, a process driven by the engine's crankshaft. The basic principle behind a naturally aspirated engine involves four key stages: intake, compression, power, and exhaust. During the intake stroke, the piston moves downward, creating a vacuum that pulls air and fuel into the cylinder through the intake valves. In the compression stroke, the intake valves close, and the piston moves upward, compressing the air-fuel mixture. The power stroke occurs when the spark plug ignites this compressed mixture, causing a small explosion that pushes the piston downward. Finally, during the exhaust stroke, the piston moves upward again, pushing exhaust gases out of the cylinder through the exhaust valves. The efficiency and performance of a naturally aspirated engine are influenced by several factors, including engine displacement, camshaft design, and valve timing. Engine displacement, measured in liters or cubic inches, determines how much air and fuel can be drawn into the cylinders. The camshaft controls the opening and closing of intake and exhaust valves, with different camshaft profiles affecting engine performance at various RPM ranges. Valve timing is critical as it ensures that air and fuel are drawn in and exhaust gases are expelled at the optimal moments. Naturally aspirated engines are known for their simplicity, reliability, and linear power delivery. They typically produce a more predictable and smoother power curve compared to forced induction engines, which can suffer from turbo lag or boost spikes. However, naturally aspirated engines generally produce less power per unit of displacement than their turbocharged or supercharged counterparts due to the limitations imposed by atmospheric pressure. In summary, naturally aspirated engines operate on the principle of using atmospheric pressure to draw air into the combustion chamber without any mechanical assistance. Their performance is optimized through careful design of engine displacement, camshaft profiles, and valve timing. While they may not offer the same level of power as forced induction engines, their simplicity and reliability make them a popular choice for many automotive applications. Understanding these principles is essential for appreciating the design and operation of naturally aspirated engines and their role in modern automotive technology.
Comparison with Forced Induction
When comparing naturally aspirated engines to those with forced induction, several key differences emerge that significantly impact performance, efficiency, and overall driving experience. Naturally aspirated engines rely solely on atmospheric pressure to draw air into the combustion chamber, which limits their power output and efficiency at higher altitudes or under heavy load conditions. In contrast, forced induction systems—such as turbochargers and superchargers—mechanically compress air before it enters the engine, allowing for a substantial increase in power and torque. **Turbocharged Engines:** Turbochargers use exhaust gases to drive a turbine that compresses intake air, thereby increasing the density of air entering the combustion chamber. This results in a significant boost in power and efficiency, especially at higher engine speeds. However, turbocharged engines often suffer from turbo lag—a delay in power delivery as the turbine spools up—though modern technologies like twin-scroll turbos and variable geometry turbines have mitigated this issue. Additionally, turbochargers can improve fuel efficiency under light load conditions by allowing the engine to run at lower boost pressures. **Supercharged Engines:** Superchargers are mechanically driven by the engine's crankshaft and compress air continuously, regardless of engine speed. This provides instant power delivery without the lag associated with turbochargers but at the cost of increased parasitic drag on the engine, which can reduce overall efficiency. Superchargers are generally simpler in design compared to turbochargers but require more power to operate, which can lead to decreased fuel economy. **Performance and Efficiency:** Forced induction allows engines to achieve higher specific power outputs than naturally aspirated counterparts. For instance, a naturally aspirated engine might produce 100 horsepower per liter of displacement, while a turbocharged or supercharged engine could easily exceed 150 horsepower per liter. However, this increased performance comes with added complexity and potential reliability concerns. Naturally aspirated engines tend to be more straightforward in design and maintenance but may lack the punch and responsiveness of their forced-induction counterparts. **Driving Experience:** The driving experience also varies significantly between naturally aspirated and forced-induction engines. Naturally aspirated engines typically offer a more linear power delivery and a more direct connection between throttle input and acceleration. Forced-induction engines, on the other hand, can provide dramatic bursts of power once the boost kicks in, making them feel more potent but sometimes less predictable. **Conclusion:** In summary, while naturally aspirated engines offer simplicity and a direct driving experience, forced-induction systems like turbochargers and superchargers provide substantial gains in power and efficiency. The choice between these technologies depends on the specific application—whether it's for everyday driving where simplicity might be preferred or for high-performance scenarios where the added complexity is justified by the significant performance gains. Understanding these differences is crucial for appreciating the unique characteristics of each type of engine and making informed decisions about which technology best suits your needs.
Historical Context and Evolution
The historical context and evolution of naturally aspirated engines are deeply intertwined with the development of internal combustion engines themselves. The journey began in the late 19th century when Nikolaus August Otto patented the first practical four-stroke engine in 1876, laying the groundwork for modern engine design. Initially, these early engines were rudimentary and inefficient but marked the beginning of a technological revolution in automotive engineering. As the early 20th century unfolded, naturally aspirated engines became the norm due to their simplicity and reliability. These engines relied solely on atmospheric pressure to draw air into the cylinders, making them straightforward to manufacture and maintain. The 1920s and 1930s saw significant improvements with advancements in materials and design, leading to more powerful and efficient engines. This period also witnessed the rise of iconic car manufacturers like Ford, General Motors, and Chrysler, who further refined naturally aspirated technology. The post-World War II era brought about a new wave of innovation, driven by advancements in materials science and aerodynamics. Engine designers began to focus on optimizing intake and exhaust systems, leading to better airflow and increased power output. The 1960s and 1970s were particularly pivotal, with the introduction of overhead camshafts, multi-valve configurations, and improved combustion chamber designs. These innovations significantly enhanced performance while maintaining the inherent simplicity of naturally aspirated engines. However, the 1980s and 1990s introduced new challenges with stringent emissions regulations and fuel efficiency standards. Engine manufacturers responded by developing more sophisticated engine management systems, including electronic fuel injection and variable valve timing. These technologies allowed naturally aspirated engines to meet regulatory requirements without sacrificing performance. In recent years, the rise of turbocharging and hybrid technologies has led some to predict the demise of naturally aspirated engines. Yet, they continue to hold a special place in automotive culture due to their unique sound, linear power delivery, and driving experience. Modern naturally aspirated engines benefit from advanced materials like aluminum and magnesium alloys, which reduce weight while maintaining strength. Additionally, advancements in computer-aided design have enabled engineers to optimize engine geometries for better efficiency and performance. Despite these advancements, naturally aspirated engines face increasing competition from forced induction alternatives that offer superior power-to-weight ratios. However, their enduring appeal lies in their mechanical simplicity, lower production costs, and the distinctive driving experience they provide. As automotive technology continues to evolve, it is clear that naturally aspirated engines will remain an integral part of automotive history and culture, even as they adapt to meet the demands of a changing world. Understanding this historical context is crucial for appreciating the significance of naturally aspirated engines in modern automotive engineering. It highlights how these engines have evolved over time to meet various challenges while retaining their core characteristics that make them so beloved by enthusiasts and engineers alike. This evolution underscores the ingenuity and innovation that have defined the automotive industry since its inception.
Key Components and Mechanics
In the intricate world of engine mechanics, several key components work in harmony to ensure optimal performance, efficiency, and reliability. At the heart of this complex system are three critical elements: the intake and exhaust systems, the camshaft and valve train, and the cylinder head design. Each of these components plays a unique role in the overall functioning of an engine. The intake and exhaust systems are responsible for managing the flow of air and fuel into the engine and expelling exhaust gases, respectively. The camshaft and valve train govern the timing and operation of the valves that control this flow. Meanwhile, the cylinder head design influences combustion efficiency and power output. Understanding these components is essential for appreciating how an engine operates effectively. By delving into these mechanics, one can gain a deeper insight into the engineering that drives modern vehicles. Let's begin by examining the **Role of Intake and Exhaust Systems**, which sets the stage for the entire engine's operation by ensuring proper airflow and gas exchange.
Role of Intake and Exhaust Systems
The role of intake and exhaust systems in a naturally aspirated engine is pivotal, as these components work in harmony to optimize the engine's performance, efficiency, and overall health. The intake system, often referred to as the induction system, is responsible for drawing air and fuel into the engine's cylinders. It typically includes the air filter, mass airflow sensor, throttle body, intake manifold, and fuel injectors. Each component plays a crucial role: the air filter cleans the air to prevent debris from entering the engine; the mass airflow sensor measures the amount of air entering the engine to ensure proper fuel-to-air ratio; the throttle body controls airflow based on driver input; and the intake manifold distributes this air-fuel mixture evenly among the cylinders. This precise delivery of air and fuel is essential for efficient combustion, which directly impacts the engine's power output and fuel efficiency. On the other side of the combustion process lies the exhaust system, which is equally vital. The exhaust system's primary function is to safely remove gases produced by combustion from the engine and direct them away from the vehicle. Key components include the exhaust valves, exhaust manifold, catalytic converter, muffler, and tailpipe. The exhaust valves open to release gases from the cylinders into the exhaust manifold, which collects these gases and directs them towards the catalytic converter. Here, harmful pollutants such as carbon monoxide, hydrocarbons, and nitrogen oxides are converted into less harmful substances like carbon dioxide, water vapor, and nitrogen. The muffler then reduces noise levels by dissipating sound energy, ensuring a quieter ride while maintaining performance. Finally, the tailpipe expels these treated gases into the atmosphere. The interplay between intake and exhaust systems is critical for maintaining optimal engine performance. For instance, a clogged air filter or faulty mass airflow sensor can lead to poor engine performance due to inadequate air supply, while a malfunctioning catalytic converter can cause increased emissions and decreased fuel efficiency. Similarly, a blocked exhaust manifold or muffler can create backpressure that hampers engine power and efficiency. Regular maintenance of these systems—such as replacing air filters and ensuring that all components are free from blockages—is essential to prevent such issues. In addition to their individual roles, intake and exhaust systems also influence each other indirectly. For example, modifications to the intake system can affect how efficiently gases are expelled through the exhaust system. Conversely, improvements in exhaust flow can enhance the engine's ability to draw in air and fuel efficiently. This symbiotic relationship underscores the importance of considering both systems when tuning or modifying an engine for better performance. In summary, the intake and exhaust systems are integral to the operation of a naturally aspirated engine. They work together to ensure that air and fuel are delivered efficiently to the cylinders for combustion and that the resulting gases are safely expelled. Proper maintenance and tuning of these systems are crucial for optimal engine performance, fuel efficiency, and environmental compliance. Understanding their mechanics and interdependencies is essential for any automotive enthusiast or mechanic looking to maximize the potential of their vehicle's engine.
Camshaft and Valve Train Functionality
In the intricate world of internal combustion engines, the camshaft and valve train are pivotal components that orchestrate the delicate dance of air and fuel intake, as well as exhaust gas expulsion. These elements are crucial for the efficient operation of a naturally aspirated engine, where air is drawn into the cylinders solely by the vacuum created by the pistons' downward motion, without any forced induction. The camshaft, a long rod with lobes of varying shapes and sizes, is the central controller of this process. As it rotates, each lobe pushes against a lifter or follower, which in turn opens and closes the valves in precise synchronization with the piston's movement. The shape and timing of these lobes determine when and how long the valves stay open, influencing engine performance characteristics such as power output, fuel efficiency, and emissions. For instance, high-performance camshafts often have more aggressive lobe profiles to allow for longer valve opening times, enhancing power at higher engine speeds. The valve train, comprising components like lifters, pushrods, rocker arms, and valves themselves, works in tandem with the camshaft to execute this valve actuation. In an overhead valve (OHV) configuration, lifters ride on the camshaft lobes and transfer this motion to pushrods that then actuate rocker arms. These rocker arms pivot to open and close the valves located in the cylinder head. In overhead camshaft (OHC) designs, the camshaft is positioned directly above the valves, eliminating the need for pushrods and allowing for more precise control over valve timing. The functionality of this system is highly dependent on precise engineering and timing. The camshaft's rotational speed is typically half that of the crankshaft due to its connection via a timing belt or chain. This ensures that valves open and close at exactly the right moments relative to piston position. For example, during the intake stroke, the intake valve must open just before the piston reaches the top dead center to allow air-fuel mixture to enter; similarly, during the exhaust stroke, the exhaust valve must open just after the piston reaches top dead center to expel gases efficiently. In naturally aspirated engines, this precise coordination between camshaft and valve train is critical because it directly affects how effectively air can be drawn into the cylinders. Without forced induction like turbocharging or supercharging, these engines rely solely on atmospheric pressure to fill their cylinders. Therefore, optimizing valve timing through camshaft design becomes a key strategy for enhancing performance without compromising fuel efficiency or reliability. In summary, the camshaft and valve train form a symbiotic relationship that is fundamental to the operation of naturally aspirated engines. By meticulously controlling when and how valves open and close, these components ensure optimal airflow and combustion efficiency, thereby influencing overall engine performance and characteristics. Understanding their functionality provides valuable insights into how naturally aspirated engines achieve their unique balance between power output and fuel economy.
Cylinder Head Design and Efficiency
Cylinder head design is a critical component in the overall efficiency and performance of an internal combustion engine, particularly in naturally aspirated engines. A naturally aspirated engine relies solely on atmospheric pressure to draw air into the cylinders, unlike turbocharged or supercharged engines which use forced induction to boost air intake. The cylinder head plays a pivotal role in this process by optimizing airflow, combustion efficiency, and heat management. Key to efficient cylinder head design is the configuration of the intake and exhaust ports. These ports must be carefully engineered to maximize airflow while minimizing resistance. Intake ports are designed to ensure a smooth, laminar flow of air-fuel mixture into the combustion chamber, often featuring curved or tapered shapes to enhance flow characteristics. Conversely, exhaust ports are optimized for efficient gas expulsion, typically with a more direct path to reduce backpressure and enhance scavenging. The combustion chamber itself is another crucial aspect of cylinder head design. Its shape and size significantly influence combustion efficiency and engine performance. A well-designed combustion chamber ensures that the air-fuel mixture is evenly distributed and that the spark plug or fuel injector is positioned for optimal ignition timing. This can include features such as a pent-roof or hemispherical design, which help in achieving a more efficient combustion process. Valve design and operation are also integral to cylinder head efficiency. The valves must open and close precisely to allow the right amount of air and fuel into the combustion chamber and exhaust gases out. Valve lift, duration, and timing are critical parameters that affect engine performance and efficiency. Modern engines often employ technologies like variable valve timing (VVT) and lift systems to dynamically adjust these parameters based on engine speed and load conditions. Cooling systems within the cylinder head are essential for maintaining optimal operating temperatures. Efficient heat dissipation prevents overheating, which can lead to engine damage or reduced performance. This is typically achieved through a combination of water jackets or air cooling channels integrated into the cylinder head design. Material selection for the cylinder head is another important factor. High-strength materials such as aluminum or cast iron are commonly used due to their excellent thermal conductivity and durability. Advanced materials like titanium or composite materials may also be employed in high-performance applications where weight reduction and enhanced strength are critical. In summary, the design of a cylinder head in a naturally aspirated engine involves meticulous attention to detail across multiple components including intake and exhaust ports, combustion chamber geometry, valve operation, cooling systems, and material selection. Each of these elements contributes to maximizing airflow efficiency, combustion effectiveness, and overall engine performance while ensuring reliable operation under various driving conditions. By optimizing these key components, engineers can create highly efficient and powerful naturally aspirated engines that deliver exceptional performance without the need for forced induction.
Performance Characteristics and Applications
When evaluating the performance characteristics and applications of modern engines, several key factors come into play. These include the power output and torque curve, which determine an engine's ability to deliver consistent and robust performance across various driving conditions. Additionally, fuel efficiency and emissions are crucial considerations, as they impact both the environmental footprint and the operational costs of the vehicle. Lastly, the versatility of an engine in different vehicle types—from compact cars to heavy-duty trucks—plays a significant role in its overall utility and market appeal. Understanding these aspects is essential for engineers, manufacturers, and consumers alike, as they influence the design, functionality, and user experience of vehicles. By examining these performance characteristics, we can better appreciate how engines are optimized for different roles and how they contribute to the broader automotive landscape. Let's begin by delving into the power output and torque curve, which form the foundation of an engine's performance profile.
Power Output and Torque Curve
When discussing the performance characteristics of an engine, particularly in the context of naturally aspirated engines, understanding power output and torque curves is crucial. Power output and torque are fundamental metrics that define an engine's performance profile. **Power output**, typically measured in horsepower (hp) or kilowatts (kW), represents the rate at which an engine can perform work. It is a function of both torque and engine speed, with higher power outputs generally indicating better acceleration and overall performance. **Torque**, on the other hand, measures the rotational force that the engine's crankshaft applies to the drivetrain. It is usually expressed in units such as pound-feet (lb-ft) or newton-meters (Nm). Torque is a key determinant of an engine's ability to haul heavy loads or accelerate from a standstill. The **torque curve** plots torque against engine speed, providing a visual representation of how torque varies across different RPM ranges. In naturally aspirated engines, which rely solely on atmospheric pressure to draw air into the cylinders without forced induction (like turbocharging or supercharging), the power output and torque curve are closely tied to engine design and tuning. For instance, a naturally aspirated engine might produce its peak torque at a lower RPM range compared to a turbocharged engine, which can generate significant torque at higher RPMs due to forced air induction. The shape of the torque curve can significantly influence an engine's usability and performance in various applications. For example, an engine with a broad, flat torque curve is often preferred in everyday driving because it provides consistent pulling power across a wide range of speeds. This makes it easier to drive in traffic and climb hills without frequent gear shifts. In contrast, engines optimized for high-performance applications may have a more peaked torque curve, delivering maximum torque at higher RPMs. This can result in exceptional acceleration but may require more frequent gear changes to stay within the optimal power band. Understanding these characteristics is essential for selecting the right engine for specific applications. For instance, in heavy-duty trucking or towing, an engine with high low-end torque is beneficial for hauling heavy loads from a standstill. In racing or high-performance driving, an engine with a high peak power output and a torque curve that peaks at higher RPMs can provide the necessary speed and agility. In summary, the power output and torque curve of a naturally aspirated engine are critical performance metrics that dictate its suitability for various applications. By analyzing these curves, engineers and enthusiasts can better understand how an engine will behave under different conditions, making informed decisions about engine tuning, vehicle design, and operational use. This nuanced understanding not only enhances performance but also ensures that the engine operates efficiently and reliably within its intended application.
Fuel Efficiency and Emissions
Fuel efficiency and emissions are critical performance characteristics that significantly impact the overall appeal and environmental footprint of naturally aspirated engines. Unlike turbocharged or supercharged engines, naturally aspirated engines rely solely on atmospheric pressure to draw air into the combustion chamber, which can influence their fuel consumption and emission profiles. In terms of fuel efficiency, naturally aspirated engines generally offer better mileage under normal driving conditions due to their simpler design and lower weight compared to forced induction systems. This simplicity reduces energy losses associated with compressing air, leading to more efficient combustion processes. However, under high-performance or high-load conditions, naturally aspirated engines may not match the fuel efficiency of turbocharged engines, which can leverage forced induction to optimize combustion at various engine speeds. Emissions from naturally aspirated engines are also a key consideration. These engines typically produce fewer emissions per unit of power output compared to their turbocharged counterparts because they operate at lower peak pressures and temperatures within the combustion chamber. Lower peak pressures reduce the formation of nitrogen oxides (NOx), a major pollutant. Additionally, the absence of turbochargers means fewer opportunities for particulate matter (PM) emissions that can arise from the combustion of fuel in high-pressure environments. Despite these advantages, modern regulations and consumer demands have driven the development of technologies that enhance both fuel efficiency and emissions control in naturally aspirated engines. For instance, advancements in engine management systems, such as direct fuel injection and variable valve timing, allow for more precise control over combustion processes, thereby optimizing fuel use and minimizing emissions. Furthermore, the integration of exhaust gas recirculation (EGR) systems helps reduce NOx emissions by recirculating a portion of the exhaust gases back into the combustion chamber. The applications of naturally aspirated engines are diverse and often tailored to specific market needs. In passenger vehicles, they are commonly found in entry-level models where cost-effectiveness and simplicity are prioritized over high-performance capabilities. In racing and high-performance applications, naturally aspirated engines are preferred for their linear power delivery and responsiveness, which are advantageous for drivers seeking precise control over engine output. In conclusion, the fuel efficiency and emissions characteristics of naturally aspirated engines make them an attractive option for various automotive applications. Their inherent simplicity contributes to better fuel economy under normal driving conditions and lower emissions compared to forced induction systems. As automotive technology continues to evolve, innovations aimed at enhancing these performance characteristics will remain crucial in meeting stringent environmental regulations while delivering satisfying driving experiences.
Use in Various Vehicle Types
In the realm of automotive engineering, naturally aspirated engines find diverse applications across various vehicle types, each leveraging their unique performance characteristics to meet specific needs. In **sports cars**, naturally aspirated engines are often preferred for their immediate throttle response and linear power delivery, which enhance the driving experience. For instance, the iconic Porsche 911 has historically featured naturally aspirated engines that provide a direct connection between the driver and the road, making it a favorite among enthusiasts. **High-performance vehicles** also benefit from naturally aspirated engines due to their ability to produce high power-to-weight ratios without the added complexity and weight of turbochargers or superchargers. The Ferrari 458 Italia, for example, utilized a naturally aspirated V8 engine that delivered exceptional power and responsiveness, contributing to its status as a benchmark in performance driving. In **racing applications**, naturally aspirated engines are frequently chosen for their reliability and simplicity. The absence of forced induction components reduces the risk of mechanical failure under extreme conditions, making them ideal for endurance racing where consistency is crucial. The Le Mans-winning Porsche 911 GT3 RSR has relied on naturally aspirated engines for their reliability and performance over long distances. **Everyday passenger vehicles** also see the use of naturally aspirated engines, particularly in smaller cars where fuel efficiency is a priority. These engines are generally lighter and less expensive to produce than their turbocharged counterparts, which can help keep vehicle costs down. For example, the Honda Civic has often featured naturally aspirated inline-four engines that balance performance with fuel economy, making it a popular choice for commuters. **Classic cars** often retain their original naturally aspirated engines as part of their nostalgic appeal. Enthusiasts appreciate the raw sound and feel of these engines, which are integral to the driving experience of vintage vehicles. The classic Ford Mustang, with its naturally aspirated V8 engine, is a prime example where the engine's character is deeply intertwined with the car's heritage. **Off-road vehicles** sometimes employ naturally aspirated engines due to their simplicity and robustness in harsh environments. The lack of complex turbocharging systems means fewer components that can fail in dusty or wet conditions, ensuring reliability when it matters most. The Land Rover Defender, known for its ruggedness, has historically used naturally aspirated engines that can withstand the rigors of off-road driving. In summary, naturally aspirated engines are versatile and find applications across a wide range of vehicle types. Their performance characteristics—such as immediate throttle response, linear power delivery, and reliability—make them suitable for everything from high-performance sports cars to everyday passenger vehicles and even off-road adventurers. Whether it's about delivering raw power or ensuring durability in challenging conditions, naturally aspirated engines continue to play a significant role in modern automotive engineering.