What Is A Joule
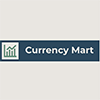
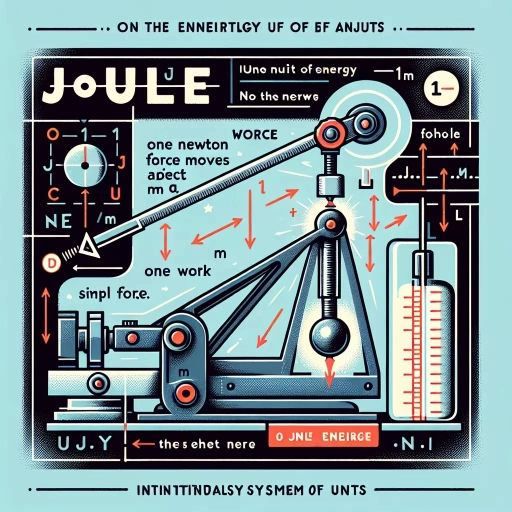
In the realm of physics, the joule is a fundamental unit of measurement that underpins our understanding of energy and its various forms. This article delves into the multifaceted nature of the joule, exploring its definition and origin, practical applications, and methods for calculating and converting it. At its core, the joule is a measure of energy that has been pivotal in scientific and engineering endeavors. To fully appreciate its significance, it is essential to understand its historical roots and the context in which it was defined. The joule's practical applications span across diverse fields, from electrical engineering to thermodynamics, making it an indispensable tool in modern technology. Additionally, knowing how to calculate and convert joules is crucial for accurate measurements and problem-solving. By examining these aspects, we gain a comprehensive insight into the joule's role in both theoretical and applied physics. Let us begin by tracing the definition and origin of the joule, a journey that will reveal the foundational importance of this unit in the scientific landscape.
Definition and Origin of the Joule
The Joule, a fundamental unit of energy in the International System of Units (SI), is a cornerstone of modern physics and engineering. This article delves into the rich history and precise definition of the Joule, providing a comprehensive understanding of its significance. We begin by exploring the **Historical Context and Naming** of the Joule, tracing its origins back to James Prescott Joule, the British physicist whose groundbreaking work in thermodynamics led to the unit's establishment. Next, we examine the **Scientific Definition and Unit** of the Joule, detailing how it quantifies energy and its role in various scientific and practical applications. Finally, we discuss the **International System of Units (SI) Recognition** of the Joule, highlighting its integration into the global standard for measurement. By understanding these facets, we gain a deeper appreciation for the Definition and Origin of the Joule.
Historical Context and Naming
The historical context and naming of the joule are deeply intertwined with the scientific advancements of the 19th century, particularly in the fields of thermodynamics and electromagnetism. The joule, named after James Prescott Joule, is a fundamental unit of energy in the International System of Units (SI). James Joule, an English physicist and brewer, was instrumental in establishing the relationship between mechanical energy and thermal energy. His groundbreaking experiments, conducted in the 1840s, led to the formulation of the first law of thermodynamics, which states that energy cannot be created or destroyed, only transformed from one form to another. Joule's work built upon earlier contributions by scientists such as Julius Robert Mayer and Hermann von Helmholtz, who also explored the concept of energy conservation. However, Joule's meticulous experiments involving the mechanical equivalent of heat—where he measured the amount of mechanical work required to produce a given amount of heat—provided empirical evidence that solidified this principle. His findings were pivotal in bridging the gap between mechanical and thermal phenomena, laying the foundation for modern thermodynamics. The term "joule" was introduced in 1889 by the British Association for the Advancement of Science (BAAS) as a tribute to James Joule's significant contributions to science. This naming was part of a broader effort to standardize units of measurement in physics, reflecting the growing need for precise and consistent terminology as scientific research expanded. The adoption of the joule as a standard unit facilitated clearer communication among scientists and engineers, enabling more accurate calculations and comparisons across various fields. In addition to its scientific significance, the naming of the joule also highlights the collaborative nature of scientific progress. Joule's work was influenced by his contemporaries, and his findings were built upon by subsequent generations of scientists. This interplay underscores how scientific discovery is often a cumulative process, with each breakthrough standing on the shoulders of previous achievements. Today, the joule is an indispensable unit in physics, engineering, and everyday life, used to measure everything from electrical energy consumption to chemical reactions. Its widespread use is a testament to James Joule's enduring legacy and the importance of his contributions to our understanding of energy and its transformations. The joule serves not only as a unit of measurement but also as a reminder of the historical context in which it was developed—a period marked by rigorous experimentation and the establishment of fundamental principles that continue to shape our understanding of the physical world.
Scientific Definition and Unit
In the realm of scientific measurement, precision and standardization are paramount. The definition and unit of a physical quantity are fundamental to understanding and communicating scientific concepts accurately. A **scientific definition** is a clear, concise, and unambiguous description of a physical quantity that distinguishes it from other quantities. It is formulated based on empirical observations, theoretical frameworks, and experimental verification. For instance, the joule, a unit of energy, is defined as the energy expended when a force of one newton is applied over a distance of one meter. This definition is rooted in the principles of mechanics and thermodynamics, ensuring that it is universally applicable and consistent across different contexts. The **unit** of a physical quantity is a specific magnitude of that quantity used as a standard for measurement. Units are essential for quantifying physical properties and facilitating comparisons between different measurements. The International System of Units (SI) provides a coherent set of units that are widely adopted across scientific disciplines. The joule, denoted by the symbol "J," is an SI unit derived from the base units of newtons and meters. It is named after James Prescott Joule, who first demonstrated the mechanical equivalent of heat in the 19th century. This historical context underscores the importance of units in bridging theoretical concepts with practical applications. The precision in defining and using units ensures that scientific measurements are reproducible and reliable. For example, when scientists measure the energy output of a device in joules, they can confidently compare their results with others who have used the same unit. This consistency is crucial for advancing scientific knowledge and technology. Moreover, the use of standardized units like the joule facilitates international collaboration and communication among researchers, engineers, and educators. In summary, the scientific definition and unit of a physical quantity like the joule are cornerstones of scientific inquiry. They provide a common language and framework for understanding and measuring physical phenomena, thereby enabling precise communication and reliable experimentation. The joule's definition as a unit of energy reflects its foundational role in physics and engineering, while its inclusion in the SI system ensures global consistency and applicability. This synergy between definition and unit underscores the rigorous methodology that underpins scientific progress.
International System of Units (SI) Recognition
The International System of Units (SI) plays a pivotal role in the global standardization of measurement, ensuring uniformity and precision across various scientific disciplines. Recognized and adopted by nearly every country, the SI system is a cornerstone of modern science and technology. Its origins date back to the French Revolution, where the need for a consistent and rational system of measurement became evident. This led to the establishment of the metric system, which was later refined and expanded into the SI system we use today. The SI system is maintained by the International Committee for Weights and Measures (ICWM) and is periodically updated to reflect advancements in science and technology. One of the fundamental units within the SI system is the joule, which is defined as the energy expended when a force of one newton is applied over a distance of one meter. This unit is crucial in fields such as physics, engineering, and chemistry, where energy calculations are paramount. The joule is named after James Prescott Joule, an English physicist who made significant contributions to the understanding of energy and its relationship to heat. His work laid the foundation for the first law of thermodynamics, which states that energy cannot be created or destroyed, only transformed from one form to another. The recognition of the joule as an SI unit underscores its importance in quantifying energy in a wide range of applications. From calculating the energy content of foods to determining the efficiency of electrical devices, the joule provides a standardized measure that facilitates accurate communication and comparison across different contexts. This standardization is essential for scientific research, industrial production, and everyday life, as it ensures that measurements are consistent and reliable. The widespread adoption of the SI system, including the joule, has been instrumental in advancing scientific knowledge and technological innovation globally. In addition to its scientific significance, the recognition of the joule highlights the collaborative effort among nations to establish a common language for measurement. This international cooperation has fostered greater precision, accuracy, and interoperability in various fields, from medicine to space exploration. As science continues to evolve, the SI system remains a vital framework that supports ongoing research and development by providing a robust and consistent foundation for measurement. The joule, as a fundamental unit within this system, continues to play an indispensable role in our understanding and application of energy principles.
Practical Applications of Joules
The concept of the joule, a fundamental unit of energy in the International System of Units (SI), has far-reaching practical applications that permeate various aspects of our daily lives and technological advancements. This article delves into the multifaceted uses of joules, exploring how they are integral to energy measurement in physics, crucial for understanding electrical energy and power systems, and essential in everyday technological and engineering applications. By examining these practical dimensions, we gain a deeper appreciation for the significance of joules in quantifying and managing energy across different fields. From the precise measurements in scientific experiments to the efficient distribution of electrical power and the innovative solutions in modern technology, the joule plays a pivotal role. This exploration will transition seamlessly into a detailed discussion on the definition and origin of the joule, providing a comprehensive understanding of this vital unit of measurement. By understanding these practical applications, we can better appreciate the foundational importance of the joule in both scientific inquiry and technological innovation.
Energy Measurement in Physics
Energy measurement in physics is a fundamental concept that underpins various practical applications, particularly when discussing the unit of energy known as the joule. The joule, named after James Prescott Joule, is a crucial metric in understanding and quantifying energy in different forms. In physics, energy measurement involves determining the amount of work that can be done by a system, which is often expressed in joules. This measurement is essential across diverse fields such as mechanics, thermodynamics, and electromagnetism. In mechanics, energy measurement helps in calculating the kinetic energy of moving objects or the potential energy stored in systems like springs or gravitational fields. For instance, when a car accelerates from rest to a certain speed, its kinetic energy increases, and this can be precisely measured in joules. Similarly, in thermodynamics, the joule is used to quantify heat transfer and internal energy changes within systems. This is vital for designing efficient engines and understanding thermal processes. Electromagnetism also relies heavily on energy measurements in joules. The electrical energy consumed by devices such as light bulbs or motors is typically measured in joules or watt-hours (where one watt-hour equals 3600 joules). This allows for accurate calculations of power consumption and efficiency, which are critical for engineering and technological advancements. Moreover, energy measurement in joules has significant practical applications in everyday life. For example, in the context of renewable energy sources like solar panels or wind turbines, understanding the output in joules helps in assessing their efficiency and overall contribution to the energy grid. In medical fields, precise energy measurements are crucial for diagnostic tools such as MRI machines and therapeutic devices like radiation therapy equipment. The precision and universality of the joule make it an indispensable unit for scientific research and technological innovation. It enables scientists to compare and analyze different types of energy across various domains, facilitating a deeper understanding of complex phenomena and driving advancements in fields ranging from materials science to aerospace engineering. In summary, energy measurement in physics, particularly through the use of joules, is a cornerstone of both theoretical understanding and practical application. It bridges the gap between abstract concepts and real-world problems, making it an essential tool for scientists, engineers, and technologists alike. By quantifying energy with such precision, we can optimize systems, improve efficiency, and innovate solutions that transform our world.
Electrical Energy and Power Systems
Electrical energy and power systems are fundamental components of modern society, leveraging the principles of joules to efficiently distribute and utilize energy. A joule, defined as the energy expended when a force of one newton is applied over a distance of one meter, serves as the cornerstone for understanding electrical power. In practical applications, electrical energy is generated through various means such as thermal, nuclear, hydroelectric, and renewable sources like solar and wind power. These sources convert mechanical or chemical energy into electrical energy, which is then transmitted over long distances via high-voltage transmission lines to minimize energy loss. The distribution of electrical energy involves a complex network of power systems, including substations, transformers, and distribution lines. Transformers play a crucial role in stepping up or stepping down voltages to optimize transmission efficiency and ensure safe delivery to consumers. For instance, high-voltage transmission lines carry electricity from power plants to substations where transformers reduce the voltage to levels suitable for residential and commercial use. This process ensures that the energy is delivered efficiently while minimizing losses due to resistance in the transmission lines. In addition to transmission and distribution, electrical power systems also incorporate advanced technologies for monitoring and control. Smart grid systems, for example, utilize real-time data analytics and IoT devices to optimize energy distribution, predict demand, and manage supply more effectively. These systems can automatically adjust voltage levels, detect faults, and reroute power to maintain reliability and reduce downtime. Moreover, the integration of renewable energy sources into traditional power grids is becoming increasingly important as societies strive for sustainability. Solar panels and wind turbines generate electricity that is fed into the grid, contributing to a cleaner energy mix. However, this integration also presents challenges such as intermittency and grid stability, which are being addressed through advancements in energy storage technologies like batteries and smart inverters. The practical applications of joules in electrical energy and power systems extend beyond generation and distribution to include consumer electronics and industrial processes. Household appliances, from refrigerators to air conditioners, operate based on the principle of converting electrical energy into mechanical or thermal energy. Industrial processes such as manufacturing and HVAC systems rely heavily on efficient electrical power to maintain productivity and safety standards. In conclusion, the concept of joules is pivotal in understanding and optimizing electrical energy and power systems. From generation through transmission and distribution to end-use applications, the efficient management of electrical energy relies on a deep understanding of joules. As technology continues to evolve, the role of joules will remain central in ensuring that electrical power systems meet the growing demands of a global population while promoting sustainability and efficiency.
Everyday Uses in Technology and Engineering
In the realm of everyday technology and engineering, the joule plays a pivotal role, underpinning numerous practical applications that shape our daily lives. One of the most ubiquitous uses of joules is in electrical power consumption. For instance, when you charge your smartphone or laptop, you are essentially converting electrical energy into joules to power these devices. The wattage of your charger, often measured in watts (joules per second), determines how quickly your device will be fully charged. Similarly, in household appliances like refrigerators and air conditioners, joules are crucial for understanding energy efficiency and consumption. Manufacturers often provide ratings in kilowatt-hours (kWh), which is a measure of joules over time, helping consumers make informed decisions about their energy usage. In transportation, joules are fundamental to understanding vehicle performance. For example, the kinetic energy of a moving car is measured in joules, reflecting the vehicle's speed and mass. This concept is essential for automotive engineers designing safer and more efficient vehicles. Additionally, electric vehicles rely on batteries that store energy in joules; the capacity of these batteries directly influences the vehicle's range and performance. In medical technology, joules are used in various diagnostic and therapeutic applications. For instance, defibrillators used to treat cardiac arrhythmias deliver electrical shocks measured in joules to restore normal heart rhythm. Similarly, laser treatments in dermatology and ophthalmology rely on precise measurements of energy in joules to ensure safe and effective procedures. In construction and civil engineering, understanding joules is critical for assessing structural integrity and safety. The impact resistance of materials like concrete and steel can be quantified in terms of joules, helping engineers design buildings that can withstand natural disasters such as earthquakes and hurricanes. Moreover, in renewable energy systems like solar panels and wind turbines, joules are used to calculate the total energy output, enabling engineers to optimize system performance and efficiency. Lastly, in consumer electronics such as speakers and headphones, sound quality is often evaluated based on power output measured in watts (joules per second). This metric helps users choose devices that meet their audio needs while ensuring they are energy-efficient. In summary, the joule is an indispensable unit of measurement across various fields of technology and engineering. From powering our daily devices to ensuring the safety and efficiency of complex systems, understanding joules is essential for innovation and practical application in modern society.
Calculating and Converting Joules
In the realm of physics, understanding energy is crucial, and one of the fundamental units of energy is the joule. Named after James Prescott Joule, who first quantified the relationship between mechanical and thermal energy, the joule is a cornerstone in measuring work and energy. This article delves into the intricacies of calculating and converting joules, providing a comprehensive guide for both students and professionals. We will explore the **Formulas for Calculating Joules**, which are essential for determining energy in various physical contexts. Additionally, we will discuss **Conversion to Other Energy Units**, enabling readers to seamlessly switch between different measurement systems. Real-world applications are also highlighted in **Real-World Examples of Joule Calculations**, demonstrating how these principles are applied in everyday life. By mastering these concepts, readers will gain a deeper understanding of energy calculations. To fully appreciate the significance of joules, it is also important to grasp their **Definition and Origin**, which we will cover in detail at the end of this article.
Formulas for Calculating Joules
When delving into the realm of energy measurement, understanding the formulas for calculating joules is paramount. A joule, the standard unit of energy in the International System of Units (SI), can be calculated using various formulas depending on the context in which energy is being measured. One of the most fundamental formulas is derived from the definition of a joule itself: **1 joule = 1 newton-meter**. This means that if you know the force applied and the distance over which it is applied, you can calculate the energy transferred using \( E = F \times d \), where \( E \) is energy in joules, \( F \) is force in newtons, and \( d \) is distance in meters. Another critical formula involves electrical energy, where joules can be calculated using the relationship between voltage, current, and time. The formula here is \( E = V \times I \times t \), where \( E \) is energy in joules, \( V \) is voltage in volts, \( I \) is current in amperes, and \( t \) is time in seconds. This formula is particularly useful in electrical engineering and electronics. For thermal energy, the formula often involves specific heat capacity and temperature change. The energy transferred due to a change in temperature can be calculated using \( E = m \times c \times \Delta T \), where \( E \) is energy in joules, \( m \) is mass in kilograms, \( c \) is specific heat capacity in joules per kilogram per degree Celsius (or Kelvin), and \( \Delta T \) is the change in temperature. In mechanical systems involving work and efficiency, the formula \( E = W \times \eta \) can be used, where \( E \) is the useful energy output in joules, \( W \) is the total work done in joules, and \( \eta \) is the efficiency of the system as a decimal value between 0 and 1. Lastly, for kinetic energy—the energy an object possesses due to its motion—the formula is \( E_k = \frac{1}{2} m v^2 \), where \( E_k \) is kinetic energy in joules, \( m \) is mass in kilograms, and \( v \) is velocity in meters per second. These formulas collectively provide a comprehensive toolkit for calculating joules across different physical contexts, ensuring that energy measurements are accurate and meaningful. By mastering these formulas, one can effectively quantify and analyze various forms of energy, which is essential in fields ranging from physics and engineering to chemistry and everyday applications. Understanding these calculations not only enhances theoretical knowledge but also facilitates practical problem-solving in real-world scenarios.
Conversion to Other Energy Units
When delving into the realm of energy measurements, understanding the conversion between different energy units is crucial for accurate calculations and comparisons. The joule, as a fundamental unit of energy in the International System of Units (SI), serves as a cornerstone for these conversions. To convert joules to other energy units, one must grasp the relationships between various forms of energy. For instance, converting joules to calories involves recognizing that 1 calorie is approximately equal to 4.184 joules. This conversion is particularly useful in nutritional contexts where energy intake is often measured in calories. Conversely, converting joules to kilowatt-hours (kWh) requires knowing that 1 kWh is equivalent to 3,600,000 joules, which is essential for understanding electrical energy consumption. Another common conversion involves joules and British Thermal Units (BTUs), where 1 BTU is roughly 1,055.056 joules. This conversion is vital in fields such as heating and cooling systems. Additionally, converting joules to electronvolts (eV) is relevant in atomic and subatomic contexts; 1 eV is approximately 1.60217662 × 10^-19 joules. The process of converting between these units often involves simple arithmetic operations based on the known conversion factors. For example, to convert a given amount of energy from joules to calories, you would divide the number of joules by 4.184. Similarly, to convert joules to kWh, you would divide by 3,600,000. Understanding these conversions not only enhances one's ability to perform calculations but also fosters a deeper appreciation for the interconnectedness of different forms of energy. Whether you are calculating the energy content of food, assessing the efficiency of an electrical appliance, or analyzing the energy levels in atomic interactions, being able to convert between various energy units seamlessly is indispensable. In practical applications, these conversions can significantly impact decision-making processes. For instance, in engineering and design, accurate energy calculations are critical for optimizing system performance and ensuring safety standards are met. In scientific research, precise conversions enable meaningful comparisons across different studies and experiments. In conclusion, mastering the art of converting joules to other energy units is a fundamental skill that underpins a wide range of scientific, engineering, and everyday applications. By understanding these relationships and performing conversions accurately, individuals can navigate complex energy-related problems with confidence and precision. This proficiency not only enhances technical capabilities but also contributes to a broader understanding of the intricate web of energy transformations that govern our world.
Real-World Examples of Joule Calculations
In the real world, joule calculations are ubiquitous and essential for understanding various phenomena and optimizing processes. For instance, in the field of electrical engineering, joules are crucial for determining the energy stored in capacitors. Consider a capacitor with a capacitance of 100 microfarads charged to 200 volts. Using the formula \(E = \frac{1}{2}CV^2\), where \(E\) is energy in joules, \(C\) is capacitance in farads, and \(V\) is voltage in volts, we can calculate the stored energy: \(E = \frac{1}{2} \times 100 \times 10^{-6} \times 200^2 = 2 \text{ joules}\). This calculation helps engineers design circuits that efficiently store and release energy. In mechanical engineering, joules are used to measure the work done by forces. For example, if a car accelerates from rest to 60 km/h over a distance of 100 meters, we can calculate the work done by the engine. Assuming an average force of 1000 newtons applied over this distance, the work done (and thus the energy expended) is given by \(W = F \times d = 1000 \times 100 = 100,000 \text{ joules}\). This helps engineers optimize engine performance and fuel efficiency. In thermodynamics, joules are vital for understanding heat transfer. When heating water from 20°C to 100°C, the energy required can be calculated using the specific heat capacity of water (approximately 4.184 joules per gram per degree Celsius). For one kilogram of water, the energy needed is \(Q = m \times c \times \Delta T = 1 \times 4.184 \times 80 = 334.72 \text{ kilojoules}\). This calculation is essential for designing heating systems and understanding thermal processes. In everyday life, joules are also relevant. For instance, when using a microwave oven to heat food, the power rating (usually in watts) can be converted into joules to understand how much energy is transferred to the food. If a microwave operates at 600 watts for 30 seconds, it delivers \(E = P \times t = 600 \times 30 = 18,000 \text{ joules}\) of energy. This helps consumers appreciate the efficiency and safety of their appliances. These examples illustrate how joule calculations permeate various fields, from engineering and thermodynamics to everyday applications. By understanding and applying these calculations, professionals and individuals can optimize systems, ensure safety, and make informed decisions about energy usage. Whether it's designing efficient electrical circuits, optimizing mechanical systems, or simply understanding how much energy goes into heating water or cooking food, joule calculations provide a fundamental framework for analyzing and managing energy in all its forms.