Why Is -40 Degrees Fahrenheit The Same As Celsius
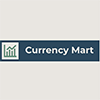
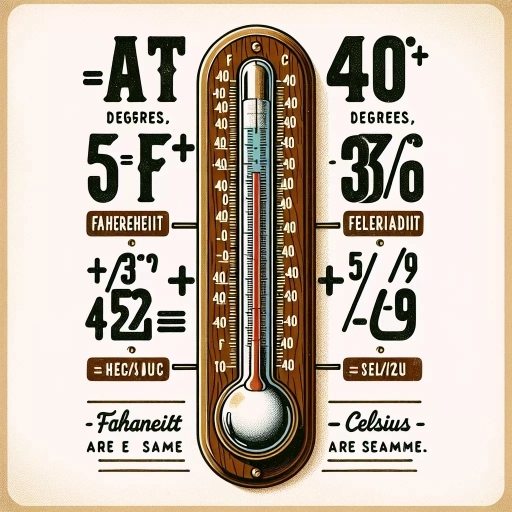
In the realm of temperature measurement, a fascinating phenomenon exists where -40 degrees Fahrenheit is identical to -40 degrees Celsius. This unique convergence is not merely a coincidence but is rooted in the fundamental principles of thermometry. To fully appreciate this intriguing fact, it is essential to delve into the underlying temperature scales, the scientific rationale behind this specific temperature point, and the practical implications it holds in real-world scenarios. This article will explore these aspects in detail, starting with an in-depth look at **Understanding the Temperature Scales**, which will lay the groundwork for comprehending how Fahrenheit and Celsius scales intersect at this critical point. By examining the historical development and mathematical structure of these scales, we can better grasp why -40 degrees Fahrenheit and -40 degrees Celsius are one and the same. This foundational understanding will then be built upon by **The Science Behind -40 Degrees**, which delves into the thermodynamic principles that make this temperature a singular point of equivalence. Finally, **Practical Implications and Real-World Examples** will illustrate how this knowledge applies in various everyday contexts, making the abstract concept tangible and relevant. Let us begin our journey by first **Understanding the Temperature Scales**.
Understanding the Temperature Scales
Understanding temperature scales is a fundamental aspect of science and everyday life, yet it often remains a subject of confusion for many. The two most widely used temperature scales, Fahrenheit and Celsius, have distinct historical origins and practical applications. In this article, we will delve into the **Historical Development of Fahrenheit and Celsius Scales**, exploring how these systems were developed and why they have endured. We will also examine the **Key Differences Between Fahrenheit and Celsius**, highlighting their unique characteristics and the contexts in which each is preferred. Additionally, we will provide **Conversion Formulas and Equivalents**, enabling readers to seamlessly switch between these scales. By grasping these concepts, you will gain a comprehensive understanding of temperature scales, enhancing your ability to navigate scientific and practical scenarios with confidence. This knowledge is essential for anyone looking to deepen their understanding of temperature scales and how they are used in various fields. Transitioning into the heart of this article, let us begin our journey to **Understanding the Temperature Scales**.
Historical Development of Fahrenheit and Celsius Scales
The historical development of the Fahrenheit and Celsius temperature scales is a fascinating narrative that reflects the evolution of scientific understanding and measurement techniques. The Fahrenheit scale, named after German physicist Gabriel Fahrenheit, was introduced in the early 18th century. In 1724, Fahrenheit developed a mercury-in-glass thermometer and defined his scale with 32 degrees as the freezing point of water and 212 degrees as the boiling point. This scale was widely adopted in the English-speaking world due to its precision and practicality for everyday applications. In contrast, the Celsius scale was developed by Swedish astronomer Anders Celsius in 1742. Initially, Celsius proposed a scale where 100 degrees was the freezing point of water and 0 degrees was the boiling point. However, after his death, French physicist Jean-Pierre Christin reversed this scale to what we know today: 0 degrees for freezing and 100 degrees for boiling. This change made the scale more intuitive and easier to use. The Celsius scale gained popularity in scientific circles because of its simplicity and logical structure. The transition from Fahrenheit to Celsius was gradual and influenced by various factors. The French Revolution played a significant role in promoting the metric system, which included the Celsius scale, as a standard for scientific and everyday use. By the mid-20th century, most countries had adopted the Celsius scale for official purposes, although Fahrenheit remained in use in some regions, particularly in the United States. Understanding these historical developments is crucial for appreciating why -40 degrees Fahrenheit is the same as -40 degrees Celsius. This unique point of equivalence occurs because both scales intersect at this specific temperature due to their respective zero points and scaling factors. Recognizing this intersection highlights the importance of understanding both scales and their historical contexts, especially in fields where precise temperature measurements are critical. In summary, the Fahrenheit and Celsius scales have distinct origins and evolutions that reflect advancements in thermometry and changes in scientific standards over time. While Fahrenheit's scale was pioneering for its era, the Celsius scale eventually became the global standard due to its logical structure and integration into the metric system. This historical backdrop underscores the significance of temperature scales in scientific inquiry and daily life, making it essential to comprehend their development and interrelation.
Key Differences Between Fahrenheit and Celsius
Understanding the nuances between Fahrenheit and Celsius temperature scales is crucial for accurate conversions and interpretations, especially when encountering temperatures like -40 degrees, which is a unique point where both scales converge. The primary difference lies in their zero points and the intervals between degrees. The Fahrenheit scale, developed by Gabriel Fahrenheit in the early 18th century, sets its zero point at the freezing point of a mixture of ice, water, and ammonium chloride, while the Celsius scale, introduced by Anders Celsius later in the same century, sets its zero point at the freezing point of pure water. This fundamental distinction means that water freezes at 32 degrees Fahrenheit but at 0 degrees Celsius. Another significant difference is the range between the freezing and boiling points of water. On the Fahrenheit scale, this range spans 180 degrees (from 32°F to 212°F), whereas on the Celsius scale, it spans 100 degrees (from 0°C to 100°C). This disparity makes Celsius more intuitive for scientific applications due to its simpler, decimal-based system. For instance, a temperature increase of 1 degree Celsius is equivalent to an increase of 1.8 degrees Fahrenheit, which can complicate calculations in the Fahrenheit system. The practical implications of these differences are evident in everyday life. Weather forecasts, for example, often use Celsius in most parts of the world due to its simplicity and ease of understanding. In contrast, Fahrenheit remains prevalent in the United States for everyday temperature readings. This dual usage can lead to confusion when converting between the two scales, especially for those not familiar with both systems. The convergence at -40 degrees is a unique point where both scales align perfectly. This occurs because -40°F is exactly -40°C, making it a convenient reference point for quick conversions. However, this alignment is an exception rather than the rule, highlighting the need for a clear understanding of each scale's characteristics to avoid errors in temperature measurements and conversions. In summary, the differences between Fahrenheit and Celsius are rooted in their historical development, zero points, and degree intervals. While Fahrenheit has historical significance and remains in use in some regions, Celsius offers a more logical and consistent system that has become the standard for scientific and international applications. Understanding these distinctions is essential for accurate temperature readings and conversions, particularly when dealing with critical temperatures like -40 degrees where both scales intersect.
Conversion Formulas and Equivalents
Understanding the intricacies of temperature scales is crucial for accurate conversions, and this involves mastering conversion formulas and equivalents. The two primary temperature scales in use today are Fahrenheit and Celsius, each with its own set of conversion formulas. To convert from Fahrenheit to Celsius, the formula is \( \text{Celsius} = \frac{5}{9} (\text{Fahrenheit} - 32) \). Conversely, to convert from Celsius to Fahrenheit, the formula is \( \text{Fahrenheit} = \frac{9}{5} (\text{Celsius}) + 32 \). These formulas are essential for everyday applications, from weather forecasting to scientific research. Another key scale is the Kelvin scale, which is used in scientific and engineering contexts. The Kelvin scale is an absolute temperature scale, meaning it has a fixed zero point that corresponds to absolute zero, the theoretical temperature at which all matter would have zero entropy. To convert from Celsius to Kelvin, you simply add 273.15: \( \text{Kelvin} = \text{Celsius} + 273.15 \). For Fahrenheit to Kelvin, you first convert Fahrenheit to Celsius using the aforementioned formula and then add 273.15. Understanding these conversion formulas is not just about memorizing equations; it's about grasping the underlying principles of each scale. For instance, the reason -40 degrees Fahrenheit is the same as -40 degrees Celsius lies in the specific design of these scales. The Fahrenheit scale was originally based on the freezing and boiling points of water, with 32 degrees being the freezing point and 212 degrees being the boiling point. The Celsius scale, on the other hand, sets these points at 0 and 100 degrees respectively. The unique intersection at -40 degrees is a result of these specific reference points and the linear nature of both scales. In practical terms, knowing these conversion formulas can be incredibly useful. For example, if you're traveling from a country that uses Celsius to one that uses Fahrenheit, being able to quickly convert temperatures can help you understand weather forecasts or set your thermostat correctly. In scientific research, precise temperature measurements are critical, and converting between scales accurately can make a significant difference in experimental outcomes. In conclusion, mastering conversion formulas between different temperature scales is fundamental for both everyday life and scientific endeavors. By understanding how to convert between Fahrenheit, Celsius, and Kelvin, you gain a deeper appreciation for the nuances of each scale and can ensure accuracy in your calculations. This knowledge not only enhances your ability to navigate different environments but also underscores the importance of precision in various fields where temperature plays a critical role.
The Science Behind -40 Degrees
In the realm of extreme temperatures, -40 degrees stands as a critical threshold that intersects multiple scientific disciplines. This temperature, where both Celsius and Fahrenheit scales converge, is more than just a numerical value; it is a gateway to understanding fundamental thermodynamic principles, the intricate behavior of molecules under extreme conditions, and the practical applications that arise from such knowledge. The science behind -40 degrees delves into the thermodynamic principles that govern how energy and matter interact at this temperature, shedding light on the concept of absolute zero and the limits of physical laws. Additionally, it explores the molecular behavior that occurs when substances are subjected to such low temperatures, revealing unique properties and phenomena. Finally, it highlights the practical applications of -40 degrees in various fields, from cryogenics to environmental science. By examining these aspects, we gain a deeper appreciation for the complex interplay of physical laws and their real-world implications, ultimately enhancing our understanding of temperature scales and their significance in scientific inquiry. Understanding the Temperature Scales becomes a natural extension of this exploration, as it underscores the importance of precise measurement and the broader context in which temperatures like -40 degrees are situated.
Thermodynamic Principles and Absolute Zero
Thermodynamic principles play a crucial role in understanding the behavior of temperatures, particularly at extreme conditions such as -40 degrees Fahrenheit, which intriguingly is the same as -40 degrees Celsius. At the heart of these principles lies the concept of absolute zero, a theoretical temperature that represents the absolute minimum possible temperature. Defined as 0 Kelvin (K), -273.15 degrees Celsius (°C), or -459.67 degrees Fahrenheit (°F), absolute zero is a fundamental benchmark in thermodynamics. It is the point at which all matter would theoretically have zero entropy, meaning all molecular motion would cease. The third law of thermodynamics states that as the temperature of a system approaches absolute zero, its entropy approaches a minimum value. However, achieving absolute zero is impossible according to the Heisenberg uncertainty principle and the third law itself, as it would require an infinite number of steps to remove all the entropy from a system. Despite this theoretical limit, scientists have been able to get very close to absolute zero through advanced techniques like laser cooling and magnetic cooling. Understanding absolute zero and the laws governing thermodynamic behavior helps explain why -40 degrees Fahrenheit and Celsius are equivalent. This unique point on the temperature scale is a result of the way the Fahrenheit and Celsius scales were historically defined. The Fahrenheit scale was developed by Gabriel Fahrenheit in the early 18th century, while the Celsius scale was introduced later by Anders Celsius. The two scales intersect at -40 degrees because of their respective zero points and the way they divide the range between the freezing and boiling points of water. In practical terms, thermodynamic principles guide our understanding of how materials behave at low temperatures. For instance, materials contract and become more brittle as they approach absolute zero, which has significant implications for engineering and materials science. Additionally, the study of thermodynamics at extreme temperatures has led to breakthroughs in fields such as cryogenics and superconductivity. In summary, the concept of absolute zero and the underlying thermodynamic principles provide a deep understanding of temperature scales and their intersections. The equivalence of -40 degrees Fahrenheit and Celsius is not just a numerical coincidence but a reflection of the historical development and mathematical structure of these scales. As scientists continue to explore temperatures near absolute zero, they uncover new phenomena that expand our knowledge of the physical world and drive technological innovation.
Molecular Behavior at Extreme Temperatures
At extreme temperatures, molecular behavior undergoes significant transformations that are crucial for understanding the unique properties of materials at such conditions. When temperatures drop to -40 degrees Fahrenheit (or Celsius), the kinetic energy of molecules diminishes dramatically. This reduction in kinetic energy leads to a substantial decrease in molecular motion, causing the molecules to move slower and come closer together. As a result, the intermolecular forces become more pronounced, influencing the physical state and properties of substances. In solids, the reduced molecular motion at -40 degrees results in increased rigidity and brittleness. The molecules are tightly packed and vibrate less, making the material more susceptible to cracking under stress. In contrast, liquids at this temperature exhibit higher viscosity due to the decreased molecular mobility, which hampers the flow of the liquid. For gases, the lower kinetic energy means that gas molecules have less energy to overcome intermolecular forces, leading to a decrease in vapor pressure and an increase in the likelihood of condensation. The behavior of water at -40 degrees is particularly noteworthy. At this temperature, water freezes into ice, but the structure of ice is such that it expands as it solidifies due to the formation of hydrogen bonds between water molecules. This expansion is why ice floats on liquid water and why pipes can burst when water inside them freezes. Moreover, at extreme low temperatures, certain materials exhibit unique properties such as superconductivity and superfluidity. For instance, some metals can become superconductors at very low temperatures, losing all electrical resistance and allowing current to flow without any loss of energy. Similarly, helium-4 becomes a superfluid at temperatures near absolute zero (-273.15 degrees Celsius), displaying zero viscosity and the ability to flow without resistance. Understanding molecular behavior at extreme temperatures is essential for various scientific and technological applications. It informs the design of materials for cryogenic storage, the operation of superconducting devices in high-energy physics experiments, and even the preservation of biological samples through cryopreservation. The intricate dance of molecules at -40 degrees Fahrenheit (or Celsius) underscores the complex interplay between temperature, molecular motion, and material properties, highlighting the fascinating science behind this critical temperature threshold.
Practical Applications of -40 Degrees
The practical applications of temperatures at -40 degrees, whether in Fahrenheit or Celsius, are diverse and critical across various fields. In meteorology, understanding this temperature threshold is essential for predicting extreme weather conditions. For instance, temperatures below -40 degrees can lead to severe frostbite and hypothermia, necessitating public health warnings and emergency preparedness measures. In aviation, pilots must be aware of such temperatures to ensure safe flight operations, as they can affect aircraft performance and safety. In the realm of materials science, -40 degrees is a benchmark for testing the durability and resilience of materials. Many industrial and automotive components are subjected to these extreme temperatures to assess their structural integrity and performance under harsh conditions. This is particularly important for industries operating in polar regions or high-altitude environments where such temperatures are common. In agriculture, knowing the impact of -40 degrees helps farmers protect their crops from devastating frost damage. Techniques such as frost protection using sprinkler systems or wind machines can be employed to mitigate damage when temperatures drop to this level. Additionally, understanding how plants respond to such extreme cold can inform breeding programs aimed at developing more resilient crop varieties. In the field of medicine, -40 degrees is used in cryopreservation techniques to preserve biological samples and tissues. This temperature is crucial for maintaining the viability of cells and tissues over extended periods, which is vital for medical research and transplantation procedures. Furthermore, in the context of energy and infrastructure, -40 degrees poses significant challenges. Pipelines and storage facilities must be designed to withstand these temperatures to prevent failures and ensure the continuous supply of essential resources like oil and natural gas. Similarly, buildings in regions prone to such cold must be insulated and constructed with materials that can endure these conditions without compromising structural integrity. Lastly, in the domain of scientific research, -40 degrees plays a critical role in various experiments. For example, in cryogenic research, scientists often work at temperatures around -40 degrees to study superconductivity and other low-temperature phenomena. These studies have led to breakthroughs in fields such as quantum computing and advanced materials science. In summary, the practical applications of -40 degrees span multiple disciplines, from public health and aviation safety to materials science, agriculture, medicine, energy infrastructure, and scientific research. Understanding and managing this temperature threshold is essential for ensuring safety, efficiency, and innovation across these diverse fields.
Practical Implications and Real-World Examples
In the realm of temperature extremes, -40 degrees stands as a critical threshold that intersects various facets of our lives, from the natural environment to industrial applications and everyday experiences. This article delves into the practical implications and real-world examples of this temperature, highlighting its significance across different domains. We will explore how weather and climate considerations play a crucial role in understanding the impact of -40 degrees on ecosystems and human activities. Additionally, we will examine the industrial and scientific uses of such extreme temperatures, revealing their importance in fields such as materials science and cryogenics. Furthermore, we will discuss everyday situations where -40 degrees matters, illustrating how this temperature affects our daily lives in more ways than we might realize. By understanding these diverse applications and implications, we can better appreciate the importance of temperature scales and their role in our world. Transitioning seamlessly into the next section, "Understanding the Temperature Scales," we will provide a comprehensive overview of how these scales are constructed and why they are essential for navigating the complexities of temperature extremes like -40 degrees.
Weather and Climate Considerations
Weather and climate considerations are crucial for understanding the practical implications of extreme temperatures, such as -40 degrees Fahrenheit, which is also -40 degrees Celsius. This unique point of equivalence highlights the importance of temperature scales in meteorology and everyday life. Weather, the short-term atmospheric condition, can significantly impact daily activities and safety. For instance, temperatures as low as -40 degrees can lead to severe frostbite within minutes, necessitating immediate protective measures like layered clothing and limited exposure. Climate, on the other hand, refers to long-term atmospheric conditions and plays a critical role in planning infrastructure, agriculture, and public health strategies. In regions where such extreme temperatures are common, buildings are designed with insulation to maintain warmth, and transportation systems are equipped with antifreeze to prevent engine failure. The practical implications of these temperatures extend beyond individual safety to economic and environmental realms. For example, in areas like Alaska or northern Canada, where -40 degrees is not uncommon during winter months, industries such as oil extraction and mining must adapt their operations to withstand these conditions. This includes using specialized equipment that can function in extreme cold and ensuring that workers have access to warm facilities during breaks. Additionally, agricultural practices must be tailored to the local climate; farmers may use greenhouses or choose crop varieties that are resilient to cold temperatures. Real-world examples illustrate these considerations vividly. In Antarctica, where temperatures frequently drop below -40 degrees Celsius, scientific research stations are built with advanced insulation and heating systems to maintain habitable conditions for researchers. Similarly, in Scandinavian countries like Sweden and Norway, public transportation systems are designed to operate efficiently even in extreme cold, ensuring that communities remain connected and functional throughout the year. Understanding the interplay between weather and climate is essential for mitigating risks associated with extreme temperatures. This knowledge informs emergency response plans, public health advisories, and infrastructure development. For instance, cities may implement cold-weather alerts to warn residents about impending dangerous conditions or invest in community warming centers to provide safe havens during prolonged cold snaps. By integrating weather and climate considerations into daily life and long-term planning, communities can better prepare for and respond to the challenges posed by temperatures like -40 degrees Fahrenheit/Celsius, ensuring safety, efficiency, and sustainability in various aspects of society.
Industrial and Scientific Uses of -40 Degrees
At -40 degrees, both Fahrenheit and Celsius scales converge, marking a unique point of equivalence. This temperature holds significant importance in various industrial and scientific applications, where precise temperature control is crucial. In the realm of materials science, -40 degrees is often used to test the durability and performance of materials under extreme conditions. For instance, automotive manufacturers subject vehicle components to -40 degrees to ensure they can withstand harsh winter conditions without compromising safety or functionality. Similarly, aerospace engineers test aircraft parts at this temperature to simulate the extreme cold encountered at high altitudes. In the field of cryogenics, -40 degrees is a critical threshold for the storage and handling of certain cryogenic fluids. While liquid nitrogen boils at -196 degrees Celsius, some cryogenic mixtures and supercooling experiments require temperatures around -40 degrees to achieve specific physical states or reactions. This temperature is also pivotal in the pharmaceutical industry for the storage of certain vaccines and biological samples that require ultra-low temperatures to maintain their potency. In environmental science, -40 degrees is used in climate modeling and weather forecasting to simulate extreme cold snaps and understand their impact on ecosystems. This helps scientists predict and prepare for severe weather events such as polar vortexes, which can have profound effects on local climates and wildlife habitats. Additionally, in the field of geology, -40 degrees is relevant for studying permafrost regions where soil and rock remain frozen year-round, influencing geological processes and ecosystems in these areas. Furthermore, in the realm of electronics and semiconductor manufacturing, -40 degrees is sometimes used to test the reliability and performance of electronic components under extreme cold conditions. This ensures that devices can operate reliably in a wide range of environmental conditions, from arctic climates to high-altitude environments. These practical implications highlight the real-world significance of -40 degrees as a benchmark for testing, research, and application across diverse fields. The convergence of Fahrenheit and Celsius at this point underscores its importance as a universal reference point in scientific and industrial contexts, facilitating international collaboration and standardization in various disciplines.
Everyday Situations Where -40 Degrees Matters
In everyday situations, the temperature of -40 degrees Fahrenheit (or Celsius) holds significant practical implications and real-world examples that underscore its critical importance. This extreme temperature marks a rare point where the Fahrenheit and Celsius scales converge, but its impact extends far beyond mere numerical curiosity. For instance, in aviation, -40 degrees is a critical threshold for aircraft operations. At this temperature, fuel viscosity increases dramatically, which can lead to engine failure if not properly managed. Pilots must take special precautions to ensure that fuel systems are adequately heated and that engines are preheated before starting, highlighting the necessity of precise temperature monitoring in aviation safety. In the automotive industry, -40 degrees poses challenges for vehicle performance and safety. Batteries lose significant capacity at such low temperatures, making it difficult to start engines. Additionally, tire pressure drops, affecting vehicle handling and increasing the risk of accidents. Car manufacturers often test their vehicles under these conditions to ensure they meet safety and performance standards, demonstrating how this temperature influences product design and consumer safety. In construction and infrastructure, -40 degrees affects the integrity of materials. Concrete curing processes are severely impacted at such low temperatures, which can lead to weakened structures if not properly managed. Builders must use specialized techniques and materials to ensure that concrete sets correctly even in extreme cold, emphasizing the importance of temperature control in construction quality. Furthermore, in medical and scientific research, -40 degrees is crucial for cryopreservation. Biological samples and pharmaceuticals are often stored at this temperature to maintain their viability and potency. Laboratories rely on precise temperature control to preserve sensitive materials, illustrating how this extreme temperature supports advancements in medical science. In everyday life, residents of regions that experience such temperatures must adapt their daily routines. For example, in places like Alaska or northern Canada, people must take extra precautions to protect themselves from hypothermia and frostbite. This includes wearing appropriate clothing, ensuring vehicles are winter-ready, and being aware of weather forecasts to avoid dangerous conditions. Lastly, in environmental monitoring and climate science, -40 degrees serves as a benchmark for understanding extreme weather patterns. Scientists study these temperatures to better comprehend climate dynamics and predict future weather trends, which is essential for developing strategies to mitigate the impacts of climate change. In summary, -40 degrees Fahrenheit (or Celsius) is not just an interesting numerical coincidence but a critical temperature with far-reaching practical implications across various sectors, from aviation and automotive to construction, medical research, everyday life, and environmental science. Understanding and managing this extreme temperature is essential for ensuring safety, performance, and progress in these fields.