Why Is Meiosis Important
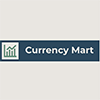
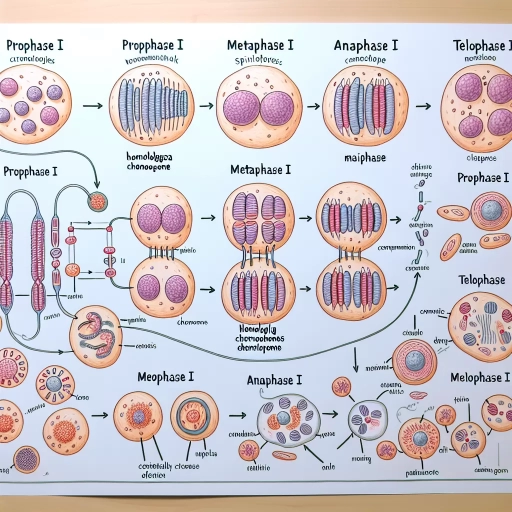
Meiosis, the intricate process of cell division that results in the production of gametes, is a cornerstone of life as we know it. This complex biological mechanism is crucial for several reasons, each highlighting its significance in different aspects of life. First, meiosis is the primary driver of genetic diversity and variation, ensuring that offspring are unique and better equipped to adapt to changing environments. Second, it plays a pivotal role in evolutionary significance, as the genetic shuffling during meiosis fuels the evolution of species by introducing new traits and combinations. Lastly, meiosis has profound biological and medical implications, influencing fertility, disease susceptibility, and our understanding of developmental biology. In this article, we will delve into these critical aspects, starting with the foundational importance of genetic diversity and variation, which sets the stage for understanding the broader impacts of meiosis on life and evolution. By exploring how meiosis generates genetic diversity, we will uncover the fundamental mechanisms that underpin the rich tapestry of life on Earth.
Genetic Diversity and Variation
Genetic diversity and variation are the cornerstone of life's resilience and adaptability, enabling species to thrive in an ever-changing environment. These concepts are intricately linked to several key processes that drive the evolution of organisms. One such process is the shuffling of genetic material, which occurs during meiosis and recombination, ensuring that offspring inherit unique combinations of genes from their parents. Another crucial mechanism is the introduction of new alleles, often through mutations or gene flow, which injects fresh genetic material into a population. This influx of new alleles can significantly enhance the genetic pool, providing the raw material for natural selection to act upon. Furthermore, genetic diversity leads to increased fitness of offspring by allowing populations to adapt more effectively to environmental challenges, thereby ensuring their survival and success. By understanding these mechanisms—shuffling of genetic material, introduction of new alleles, and increased fitness of offspring—we can gain a deeper insight into the complex and dynamic nature of genetic diversity and variation. This article will delve into these supporting ideas, exploring how they collectively contribute to the rich tapestry of genetic diversity and variation.
Shuffling of Genetic Material
Shuffling of genetic material is a pivotal process that significantly contributes to genetic diversity and variation, making it a cornerstone of meiosis. During meiosis, specifically in the prophase I stage, homologous chromosomes undergo a series of intricate steps that lead to the exchange of genetic material. This process, known as crossing over, involves the physical breakage and rejoining of DNA strands between non-sister chromatids of homologous chromosomes. As a result, new combinations of alleles are formed, enhancing genetic diversity by increasing the likelihood that offspring will inherit unique combinations of traits. Another critical mechanism involved in the shuffling of genetic material is independent assortment. During the metaphase I stage of meiosis, homologous pairs of chromosomes align at the metaphase plate in a random manner. When these chromosomes separate during anaphase I, each pole receives a random assortment of chromosomes, further increasing genetic variation. This randomness ensures that each gamete (sperm or egg cell) produced is genetically distinct from others, even if they come from the same individual. The cumulative effect of crossing over and independent assortment is profound. These mechanisms ensure that the genetic makeup of offspring is not a simple replication of parental traits but rather a unique blend that can include new trait combinations not seen in either parent. This genetic shuffling is essential for several reasons: it allows populations to adapt more readily to changing environments by providing a diverse gene pool from which natural selection can act; it increases the likelihood of beneficial mutations being expressed; and it helps to maintain the health of populations by reducing the incidence of deleterious recessive alleles through heterozygosity. In addition, the shuffling of genetic material during meiosis plays a crucial role in maintaining species integrity. By ensuring that each generation has a diverse set of genetic traits, meiosis helps to prevent the accumulation of harmful mutations and promotes evolutionary innovation. This diversity is particularly important in sexually reproducing organisms, where it allows for the creation of new phenotypes that can better survive and reproduce in varying environmental conditions. In summary, the shuffling of genetic material through crossing over and independent assortment during meiosis is fundamental to generating genetic diversity and variation. This process underpins the importance of meiosis in ensuring that populations remain adaptable, resilient, and capable of evolving over time. By fostering a rich tapestry of genetic combinations, meiosis ensures that life can thrive in an ever-changing world.
Introduction of New Alleles
The introduction of new alleles is a pivotal process that significantly enhances genetic diversity and variation within populations. This phenomenon is largely facilitated by meiosis, the complex and highly regulated form of cell division that occurs during the production of gametes (sperm and egg cells). During meiosis, genetic material is shuffled through mechanisms such as crossing over and independent assortment, leading to the creation of unique combinations of alleles in each gamete. Crossing over, for instance, involves the exchange of segments between homologous chromosomes, resulting in recombinant chromosomes that carry new allele combinations not present in the parent cells. This process breaks the linkage between alleles, allowing for greater genetic recombination and increasing the likelihood that offspring will inherit novel allele combinations. Independent assortment further contributes to this diversity by randomly distributing chromosomes into gametes, ensuring that each gamete has a unique set of alleles. The introduction of new alleles can also occur through mutations, which are changes in the DNA sequence of an individual. These mutations can arise spontaneously during DNA replication or be induced by environmental factors such as radiation and chemicals. While many mutations may be neutral or deleterious, some can confer advantageous traits that enhance an individual's fitness and survival capabilities. When these beneficial mutations are passed on to subsequent generations, they contribute to the pool of genetic variation within a population. Moreover, genetic drift and gene flow play crucial roles in the distribution and introduction of new alleles within populations. Genetic drift refers to the random change in allele frequencies over time, which can lead to the fixation of new alleles in a population. Gene flow, on the other hand, involves the movement of individuals with different alleles into a new population, thereby introducing genetic variation that was previously absent. In summary, the introduction of new alleles is a multifaceted process driven by meiotic recombination, mutations, genetic drift, and gene flow. These mechanisms collectively enrich the genetic landscape of a population, providing the raw material for natural selection to act upon. This increased genetic diversity is essential for the adaptability and resilience of populations in changing environments, underscoring why meiosis is so important for maintaining and enhancing biodiversity. By ensuring that each generation inherits a unique set of alleles, meiosis fosters evolutionary potential and supports the long-term survival of species.