Why Does Atomic Radius Decrease From Left To Right
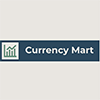
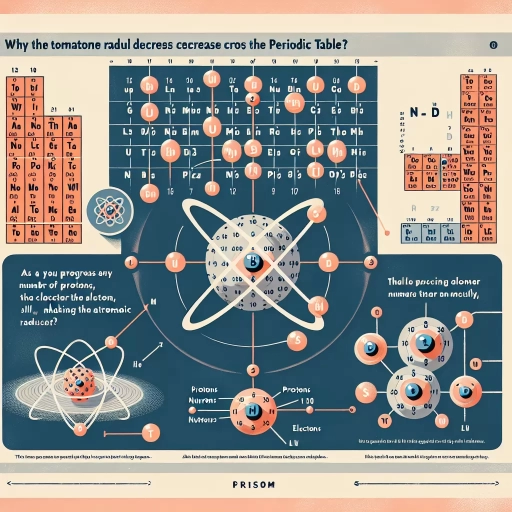
The atomic radius, a fundamental property in chemistry, exhibits a fascinating trend across the periodic table: it decreases from left to right within a period. This phenomenon is not merely an observational curiosity but is deeply rooted in the underlying principles of atomic structure and electron configuration. To understand why this trend occurs, it is essential to delve into several key aspects. First, we must **Understand the Periodic Trend of Atomic Radius**, which sets the stage for comprehending the broader pattern. Next, we need to explore **The Role of Electron Configuration in Atomic Radius Decrease**, as the arrangement of electrons significantly influences the size of an atom. Finally, we must consider **Factors Influencing the Decrease in Atomic Radius Across a Period**, including the effects of nuclear charge and electron shielding. By examining these elements, we can gain a comprehensive insight into why atomic radius diminishes as we move from left to right across a period. Let us begin by **Understanding the Periodic Trend of Atomic Radius** to lay the groundwork for our detailed analysis.
Understanding the Periodic Trend of Atomic Radius
Understanding the periodic trend of atomic radius is a fundamental concept in chemistry, as it reveals how the size of atoms changes across the periodic table. This trend is influenced by several key factors, each contributing to the overall pattern observed. First, the electron shell configuration plays a crucial role, as the arrangement of electrons in different energy levels affects the atomic radius. Second, the effective nuclear charge, which is the net positive charge experienced by an electron, significantly impacts the size of an atom. Lastly, the shielding effect, where inner electrons shield outer electrons from the full force of the nuclear charge, also plays a vital role in determining atomic radius. By examining these three aspects—electron shell configuration, effective nuclear charge, and shielding effect—we can gain a comprehensive understanding of why atomic radii vary systematically across the periodic table. This article will delve into each of these factors, providing a detailed exploration of how they collectively shape the periodic trend of atomic radius.
Electron Shell Configuration and Atomic Radius
Understanding the periodic trend of atomic radius is intricately linked to electron shell configuration, a fundamental concept in chemistry. The atomic radius, which is the distance from the nucleus to the outermost electron in an atom, varies systematically across the periodic table due to changes in electron shell configurations. As you move from left to right within a period, the atomic radius generally decreases. This trend can be explained by examining how electrons are arranged in their shells and the effective nuclear charge. In an atom, electrons occupy specific energy levels or shells, each with its own set of subshells. The principal quantum number (n) defines the energy level, while the azimuthal quantum number (l) specifies the subshell within that level. As you traverse a period from left to right, electrons are added to the same principal energy level but to different subshells. For instance, in the second period, electrons start filling the 2s and then the 2p subshells. The key factor influencing atomic radius is the effective nuclear charge, which is the net positive charge experienced by an electron in an atom. As you move across a period, protons are added to the nucleus, increasing the nuclear charge. However, the electrons added to the same principal energy level do not shield each other effectively from this increased nuclear charge. This results in a stronger attraction between the nucleus and the outermost electrons, pulling them closer to the nucleus and thus reducing the atomic radius. Additionally, when transitioning from s to p subshells within the same period, there is no significant increase in shielding effect because these electrons are not very effective at shielding each other due to their different orbital shapes and orientations. Consequently, as more protons are added without a corresponding increase in shielding, the effective nuclear charge increases relative to the number of electrons in the outermost shell, leading to a contraction of the atomic radius. This systematic decrease in atomic radius from left to right within a period is a direct consequence of these electronic and nuclear interactions. It underscores how fundamental principles such as electron shell configuration and effective nuclear charge govern periodic trends in atomic properties, providing a coherent framework for understanding chemical behavior across different elements. By grasping these concepts, chemists can predict and explain various chemical phenomena with greater precision and accuracy.
Effective Nuclear Charge and Its Impact
Effective nuclear charge is a critical concept in understanding the periodic trends of atomic radius, particularly the decrease observed from left to right across a period. Effective nuclear charge refers to the net positive charge experienced by an electron in an atom, which is the actual nuclear charge minus the shielding effect of inner electrons. As you move from left to right across a period, the atomic number increases, meaning there are more protons in the nucleus. However, the number of electron shells remains constant within the same period. This results in an increase in the effective nuclear charge because each additional proton adds to the overall positive charge without a corresponding increase in shielding by inner electrons. The impact of this increasing effective nuclear charge is profound. It leads to a stronger attraction between the nucleus and the outermost electrons, pulling them closer to the nucleus. Consequently, the atomic radius decreases as the electrons are drawn inward due to the enhanced electrostatic pull. This trend is consistent across each period of the periodic table, where elements on the left have larger atomic radii compared to those on the right. Moreover, the increase in effective nuclear charge also influences other chemical properties such as electronegativity and ionization energy. Electronegativity, which is the ability of an atom to attract electrons in a covalent bond, increases from left to right due to the stronger nuclear pull on electrons. Similarly, ionization energy—the energy required to remove an electron from an atom—also increases because it becomes more difficult to detach an electron from an atom with a higher effective nuclear charge. Understanding effective nuclear charge is essential for predicting and explaining various chemical behaviors and trends. For instance, it helps in explaining why elements on the right side of the periodic table tend to form anions more readily than those on the left. The increased effective nuclear charge makes it easier for these elements to gain electrons and form stable anions. In summary, the concept of effective nuclear charge provides a fundamental explanation for why atomic radius decreases from left to right across a period. The increasing positive charge experienced by outer electrons due to additional protons without corresponding shielding leads to a stronger nuclear attraction, resulting in smaller atomic radii. This principle underpins many periodic trends and is crucial for understanding atomic and chemical properties.
Shielding Effect and Its Role in Atomic Radius
The shielding effect plays a crucial role in understanding the periodic trend of atomic radius, particularly why atomic radius decreases from left to right across a period. This phenomenon is rooted in the way electrons are arranged and interact within an atom. The shielding effect refers to the reduction in the attractive force between the nucleus and an electron due to the presence of inner electrons. These inner electrons shield the outer electrons from the full nuclear charge, effectively reducing the nuclear pull on them. As you move from left to right across a period in the periodic table, the atomic number increases, meaning more protons are added to the nucleus. This increase in nuclear charge would intuitively suggest that the atomic radius should decrease due to a stronger pull on the electrons. However, it's not just about the number of protons; it's also about how effectively these protons are shielded by inner electrons. In the early elements of a period, the electrons are added to the same principal energy level (or shell), but they do not significantly shield each other because they are in the same energy level and have similar shielding effects. As a result, each additional proton in the nucleus exerts a greater effective nuclear charge on the outermost electrons, pulling them closer to the nucleus. This increased effective nuclear charge outweighs any minor shielding effects from the same energy level electrons, leading to a decrease in atomic radius. Moreover, as you progress across a period, the number of electron shells remains constant, but the number of electrons in those shells increases. Since these additional electrons are in the same principal energy level and do not provide significant shielding, they do not counteract the increased nuclear charge effectively. Consequently, the overall effect is a stronger nuclear pull on each electron, resulting in a tighter binding and a smaller atomic radius. In summary, the shielding effect is pivotal in explaining why atomic radius decreases from left to right across a period. The inability of electrons within the same energy level to shield each other effectively from the increasing nuclear charge leads to a stronger attraction between the nucleus and outer electrons, thereby reducing the atomic radius. This trend underscores the complex interplay between nuclear charge and electron shielding in determining atomic size.
The Role of Electron Configuration in Atomic Radius Decrease
The atomic radius, a fundamental property of elements, undergoes significant changes across the periodic table, a phenomenon largely influenced by electron configuration. Understanding the role of electron configuration in the decrease of atomic radius is crucial for grasping the periodic trends that govern chemical behavior. This article delves into three key aspects that contribute to this decrease: the influence of valence electrons on atomic size, the effects of subshell filling on radius, and the impact of electron-electron repulsion. By examining how valence electrons shape the outermost shell of an atom, we can see how their arrangement affects overall size. Additionally, the filling of subshells introduces complexities that alter atomic dimensions. Finally, electron-electron repulsion plays a significant role in compressing the electron cloud, further reducing the atomic radius. These factors collectively explain why atomic radii tend to decrease across periods and down groups in the periodic table, providing a comprehensive understanding of the periodic trend of atomic radius.
Valence Electrons and Their Influence on Size
Valence electrons play a pivotal role in determining the size of an atom, particularly when examining the trend of atomic radius decrease from left to right across a period in the periodic table. These outermost electrons are crucial because they are involved in chemical bonding and directly influence the overall size of an atom. As you move from left to right within a period, the number of protons in the nucleus increases, leading to a stronger nuclear pull on the electrons. This increased nuclear charge results in a tighter binding of the valence electrons to the nucleus, causing them to be drawn closer and reducing the atomic radius. The key factor here is the effective nuclear charge, which is the net positive charge experienced by the valence electrons. As more protons are added to the nucleus, this effective nuclear charge increases, despite the addition of electrons to the same principal energy level. The electrons added to the same shell do not shield each other effectively from the increasing nuclear charge, leading to a more significant pull on each electron. Consequently, the distance between the nucleus and the valence electrons decreases, resulting in a smaller atomic radius. Furthermore, the electron configuration also plays a role in this trend. As you move across a period, electrons are added to the same principal energy level but to different subshells. For instance, in the second period, electrons are added to the 2s and 2p subshells. The 2p subshells are higher in energy than the 2s subshell but are still within the same principal energy level. The electrons in these subshells experience a similar effective nuclear charge, which increases as you move across the period. This consistent increase in effective nuclear charge without significant shielding by additional electrons leads to a systematic decrease in atomic radius. In summary, the influence of valence electrons on atomic size is primarily through their interaction with the increasing nuclear charge as you move from left to right across a period. The tighter binding of these electrons due to the enhanced effective nuclear charge results in a decrease in atomic radius. Understanding this relationship is essential for grasping why atomic radius decreases across a period and how electron configuration contributes to this phenomenon. This fundamental concept underpins many chemical properties and behaviors of elements, making it a cornerstone of atomic physics and chemistry.
Subshell Filling and Its Effects on Radius
As we delve into the intricacies of atomic structure and its impact on atomic radius, the concept of subshell filling emerges as a pivotal factor. The arrangement of electrons within an atom, governed by the Aufbau principle and the Pauli exclusion principle, dictates how electrons occupy various subshells. When considering the trend of decreasing atomic radius from left to right across a period in the periodic table, the manner in which subshells are filled plays a crucial role. In the context of electron configuration, each subshell has a specific capacity for electron occupancy. For instance, the s subshell can hold up to 2 electrons, while the p subshell can accommodate up to 6 electrons. As we move from one element to the next within a period, additional electrons are added to the same principal energy level but to different subshells or orbitals. This incremental addition of electrons does not significantly increase the atomic radius because these electrons are being added to the same energy level, which does not extend the overall size of the atom. Moreover, the effective nuclear charge increases as we move from left to right across a period due to the addition of protons in the nucleus without a corresponding increase in the number of energy levels. This heightened nuclear charge pulls the electrons closer to the nucleus, resulting in a contraction of the atomic radius. The filling of subshells, particularly the p subshells which are less effective at shielding nuclear charge compared to s electrons, exacerbates this effect. Each additional electron in the p subshell experiences a greater effective nuclear charge, leading to a tighter binding and thus a smaller atomic radius. Additionally, the electron-electron repulsion within the same energy level also influences atomic size. As more electrons are added to the same principal energy level, they occupy different orbitals but still interact with each other. This repulsion can slightly counteract the effect of increased nuclear charge, but it is generally overshadowed by the dominant effect of nuclear attraction. In summary, the filling of subshells and the subsequent increase in effective nuclear charge are key drivers behind the decrease in atomic radius from left to right across a period. Understanding these principles provides insight into why atoms become smaller as we traverse the periodic table horizontally, highlighting the intricate interplay between electron configuration and atomic size. This phenomenon underscores the importance of electron configuration in determining fundamental atomic properties and their trends across the periodic table.
Electron-Electron Repulsion and Its Contribution
Electron-electron repulsion plays a pivotal role in understanding the decrease in atomic radius as one moves from left to right across a period in the periodic table. This phenomenon is intricately linked with electron configuration, which dictates how electrons are arranged within an atom. As atoms gain electrons in the same principal energy level (or shell), these additional electrons occupy the same orbital type, leading to increased electron-electron repulsion. This repulsion arises because like charges (in this case, negatively charged electrons) naturally repel each other. However, despite this repulsion, the effective nuclear charge—the net positive charge experienced by an electron—increases due to the addition of protons in the nucleus as one moves across a period. The rise in effective nuclear charge pulls the electrons closer to the nucleus, counteracting the repulsive forces between them. The interplay between these two forces is crucial: while electron-electron repulsion tends to expand the atom by pushing electrons apart, the increased effective nuclear charge acts to contract it by pulling electrons closer. In most cases, the latter effect dominates, resulting in a decrease in atomic radius. This contraction is further exacerbated by the fact that electrons added across a period are placed in the same principal energy level, where they experience a stronger attraction to the nucleus compared to electrons in higher energy levels. Consequently, as atoms gain more electrons and protons across a period, their atomic radii generally decrease due to this balance of forces. Understanding electron-electron repulsion and its contribution to atomic radius trends is essential for grasping various chemical properties and behaviors. For instance, it influences ionization energy and electronegativity, which are critical in predicting how atoms interact with each other to form molecules. The decrease in atomic radius also affects the reactivity of elements, as smaller atoms tend to have higher ionization energies and are less likely to lose electrons easily. Thus, recognizing the role of electron-electron repulsion in shaping atomic dimensions provides a fundamental insight into the periodic trends that underpin much of chemistry and physics. By appreciating this intricate balance of forces within an atom, scientists can better explain and predict a wide range of chemical phenomena, making it a cornerstone concept in understanding the periodic table's structure and its implications for chemical behavior.
Factors Influencing the Decrease in Atomic Radius Across a Period
The atomic radius, a fundamental property in chemistry, exhibits a notable trend across a period in the periodic table: it decreases. This phenomenon is not merely a curiosity but a reflection of several underlying factors that influence atomic structure. As we move from left to right across a period, the increasing proton number and effective nuclear charge play a crucial role in pulling electrons closer to the nucleus, thereby reducing the atomic radius. Additionally, the constant energy level at which electrons are added across a period means that these electrons are drawn into a smaller space, contributing to the decrease in radius. Furthermore, the increase in electronegativity across a period correlates with the reduction in atomic size, as atoms become more effective at attracting electrons. Understanding these factors—increasing proton number and effective nuclear charge, constant energy level and decreasing radius, and electronegativity and its correlation with atomic size—is essential for grasping the periodic trend of atomic radius. By delving into these concepts, we can gain a deeper insight into why atomic radii decrease across a period, enhancing our comprehension of the periodic table's underlying principles.
Increasing Proton Number and Effective Nuclear Charge
As we traverse a period from left to right in the periodic table, one of the key factors influencing the decrease in atomic radius is the increase in proton number and the corresponding rise in effective nuclear charge. This phenomenon is rooted in the fundamental principles of atomic structure and electron configuration. When moving across a period, each subsequent element gains one proton in its nucleus, thereby increasing the overall positive charge. This increment in proton number enhances the attractive force exerted by the nucleus on the electrons, particularly those in the outermost shell. The effective nuclear charge, which is the net positive charge experienced by an electron, also increases due to the addition of protons. Although electrons are added to the same principal energy level (or shell) as we move across a period, these electrons do not shield each other effectively because they occupy different orbitals within that shell. As a result, each additional proton adds to the effective nuclear charge without being significantly counterbalanced by electron shielding. This heightened effective nuclear charge pulls the electrons closer to the nucleus, reducing the distance between the nucleus and the outermost electrons. Consequently, the atomic radius decreases as we move from left to right across a period. This contraction is evident in various physical and chemical properties of elements, such as their ionization energies and electronegativities, which generally increase across a period due to the stronger nuclear pull on electrons. Furthermore, this trend is consistent with the periodic trends observed in other properties like electron affinity and metallic character, which also reflect changes in atomic size and electron-nucleus interactions. The interplay between increasing proton number and effective nuclear charge thus forms a critical basis for understanding why atomic radii systematically decrease across a period, providing a coherent explanation for these observed trends in the periodic table. This fundamental concept underscores the importance of nuclear-electron interactions in shaping the periodic behavior of elements.
Constant Energy Level and Decreasing Radius
As we traverse the periodic table from left to right within a period, a significant trend emerges: the atomic radius decreases. This phenomenon is intricately linked to the concept of constant energy levels and the interplay between electron shielding, nuclear charge, and orbital penetration. When electrons occupy the same principal energy level (n), they experience a constant overall energy environment. However, as we move across a period, the atomic number increases, leading to an augmentation in the nuclear charge. This heightened positive charge at the nucleus exerts a stronger pull on the electrons, drawing them closer to the nucleus. Despite the addition of electrons to the same energy level, which might suggest an increase in radius due to electron-electron repulsion, the dominant effect is the increased nuclear attraction. Electron shielding, which is the ability of inner electrons to shield outer electrons from the full effect of the nuclear charge, remains relatively constant within the same energy level. However, as more protons are added to the nucleus without a corresponding increase in shielding electrons, each subsequent electron added to the same energy level experiences a greater effective nuclear charge. This results in a tighter binding of electrons to the nucleus, leading to a decrease in atomic radius. Furthermore, orbital penetration plays a crucial role. Electrons in lower energy levels penetrate closer to the nucleus than those in higher energy levels due to their higher probability density near the nucleus. As we move across a period, electrons are added to the same principal energy level but with different orbital types (s, p, d). The s-orbitals penetrate more effectively than p-orbitals, which in turn penetrate more than d-orbitals. However, since we are dealing with the same principal energy level across a period, this effect is less pronounced compared to the increasing nuclear charge. In summary, the decrease in atomic radius across a period is primarily driven by the increase in nuclear charge without a corresponding increase in electron shielding. This results in a stronger attraction between the nucleus and electrons, pulling them closer and reducing the atomic radius. Understanding these factors provides a clear insight into why atomic radii diminish as we move from left to right within a period on the periodic table.
Electronegativity and Its Correlation with Atomic Size
Electronegativity, a measure of an atom's ability to attract electrons in a covalent bond, plays a crucial role in understanding the trends in atomic size across a period. As we move from left to right in the periodic table, the atomic radius decreases, and this phenomenon is closely correlated with the increase in electronegativity. Electronegativity rises due to the effective nuclear charge, which is the net positive charge experienced by an electron in an atom. This increase in effective nuclear charge is a result of the addition of protons to the nucleus without a corresponding increase in the number of electron shells, leading to a stronger pull on the electrons. As electronegativity increases, the atoms become more efficient at attracting electrons towards themselves. This heightened attraction results in a tighter hold on the electrons, causing them to be drawn closer to the nucleus. Consequently, the atomic radius decreases because the distance between the nucleus and the outermost electrons diminishes. This effect is particularly pronounced across a period where the same principal energy level is being filled, and each subsequent element adds one more proton to the nucleus, enhancing the nuclear pull. Moreover, the increase in electronegativity also influences the formation of bonds and the overall electron configuration of atoms. In molecules, higher electronegativity values indicate that an atom will pull shared electrons closer, potentially leading to shorter bond lengths and more compact molecular structures. This compactness is a direct reflection of the reduced atomic radius due to increased electronegative forces. The interplay between electronegativity and atomic size is further underscored by the fact that elements with high electronegativity values tend to form ions with smaller radii. For instance, fluorine, which has the highest electronegativity among all elements, forms ions with very small radii compared to other elements in its period. This correlation highlights how electronegativity not only affects atomic size but also influences ionic radii and overall chemical behavior. In summary, the increase in electronegativity across a period is a key factor contributing to the decrease in atomic radius. The enhanced effective nuclear charge and subsequent stronger attraction of electrons towards the nucleus result in smaller atomic radii. Understanding this relationship is essential for predicting chemical properties and behaviors of elements and their compounds, making it a fundamental concept in chemistry.