Why Can't Penguins Fly
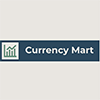
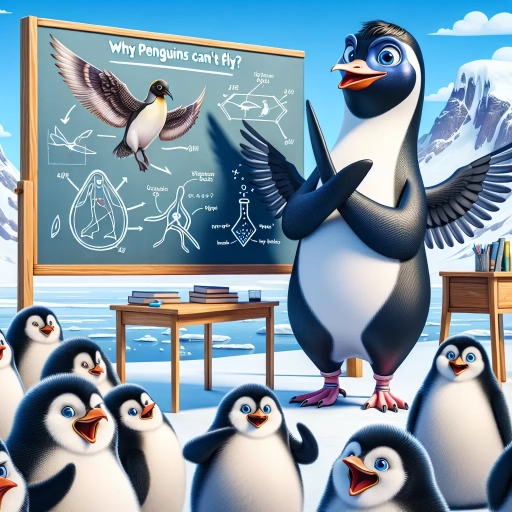
Penguins, with their distinctive waddling gait and agile swimming abilities, are one of the most fascinating creatures in the animal kingdom. However, despite their remarkable adaptations for aquatic life, penguins are unable to fly—a trait that sets them apart from their avian counterparts. The reasons behind this inability are multifaceted and deeply rooted in various biological and evolutionary factors. This article delves into the three primary reasons why penguins cannot fly: evolutionary trade-offs, anatomical limitations, and physiological constraints. By examining these aspects, we can gain a deeper understanding of how penguins' unique adaptations have shaped their survival and success in their marine environment. First, we will explore the evolutionary trade-offs that have led penguins to prioritize swimming over flying, highlighting how these choices have influenced their overall biology and lifestyle. This foundational understanding will set the stage for a comprehensive analysis of the anatomical and physiological factors that further explain why penguins remain flightless. Let us begin by examining the evolutionary trade-offs that have defined the penguin's flightless existence.
Evolutionary Trade-Offs
In the intricate dance of evolution, organisms often face a series of trade-offs that shape their survival and success. These trade-offs are particularly evident in species that undergo significant adaptations to new environments, such as the transition from terrestrial to aquatic habitats. For instance, when species adapt to aquatic environments, they must balance various physiological and structural changes. One key aspect is the enhancement of energy efficiency in swimming, which can come at the cost of other physical attributes. Additionally, body structure modifications are crucial for navigating water effectively, yet these changes may compromise performance in other areas. This article delves into these specific adaptations—adaptation to aquatic environments, energy efficiency in swimming, and body structure modifications—to illustrate how these evolutionary trade-offs influence the evolution of species. By examining these trade-offs, we gain a deeper understanding of the complex interplay between environmental pressures and biological adaptations, ultimately revealing the multifaceted nature of evolutionary trade-offs.
Adaptation to Aquatic Environment
Adaptation to the aquatic environment is a fascinating example of evolutionary trade-offs, particularly evident in the case of penguins. These flightless birds have evolved remarkable adaptations that enable them to thrive in their marine habitat, but these advancements come at the cost of their ability to fly. One of the key adaptations is their streamlined body shape, which reduces drag and enhances swimming efficiency. Their wings, though not suited for flight, have transformed into powerful flippers that propel them through the water with remarkable agility and speed. The dense, oily feathers of penguins provide insulation against cold water temperatures, while their unique feather structure allows them to maintain a layer of air next to their skin for additional warmth. Another critical adaptation is their visual system. Penguins have eyes that are adapted for underwater vision, with a reflective layer called the tapetum lucidum that enhances light sensitivity in low-light conditions. This adaptation is crucial for hunting fish and other aquatic prey. Additionally, penguins have a highly efficient respiratory system that allows them to conserve oxygen while diving deep into the ocean. Their lungs are more efficient at extracting oxygen from the air, and their muscles contain high levels of myoglobin, which stores oxygen for use during prolonged dives. However, these adaptations come with significant trade-offs. The energy and resources invested in developing strong flippers and a streamlined body mean that penguins lack the lightweight bones, hollow skeletons, and powerful chest muscles necessary for flight. Their feathers, though ideal for insulation in water, are not light enough or structured in a way that would allow them to generate lift in the air. Furthermore, the metabolic demands of swimming and diving are high, leaving less energy available for other activities such as flying. In summary, the adaptation of penguins to their aquatic environment is a prime example of evolutionary trade-offs. While their unique physical and physiological traits make them highly successful in their marine habitat, these same traits render them incapable of flight. This trade-off highlights the principle that evolutionary adaptations often involve compromises, where gains in one area come at the expense of others. Understanding these trade-offs provides valuable insights into the complex processes that shape the evolution of species and their environments.
Energy Efficiency in Swimming
Energy efficiency in swimming is a critical aspect of evolutionary trade-offs, particularly evident in species like penguins, which have adapted to aquatic environments at the expense of flight capabilities. Penguins exemplify this trade-off through their unique physiological and anatomical adaptations that optimize energy expenditure while swimming. One key adaptation is their streamlined body shape, which reduces drag and allows them to glide through the water with minimal resistance. Their wings, though not suited for flying, have evolved into powerful flippers that propel them forward with great efficiency. The dense feathers and thick layer of fat (blubber) provide insulation, reducing heat loss and conserving energy in cold water environments. Moreover, penguins' skeletal system is highly specialized; their bones are solid rather than hollow, which adds weight but also provides the necessary ballast for diving deep into the ocean. This adaptation, combined with their ability to slow down their heart rate and reduce blood flow to non-essential organs during dives, helps in conserving oxygen and thus energy. Additionally, penguins employ efficient swimming techniques such as "wing-propelled diving," where they use their flippers to generate thrust, and "tobogganing," where they slide on their bellies to reduce friction and conserve energy on land. The evolutionary trade-offs that penguins have made for enhanced swimming efficiency are starkly contrasted with their inability to fly. The energy saved from not having to maintain flight capabilities is redirected towards developing strong muscles and efficient swimming mechanisms. This specialization highlights the principle of evolutionary trade-offs, where improvements in one trait often come at the expense of another. In the case of penguins, their remarkable aquatic prowess is a direct result of sacrificing aerial abilities, demonstrating how species adapt to their environments in ways that maximize their survival and reproductive success. Understanding these trade-offs provides valuable insights into the complex interplay between different traits and how they influence an organism's overall fitness. For instance, while penguins may not be able to soar through the skies like their flying relatives, their extraordinary swimming abilities allow them to thrive in their aquatic habitat. This dichotomy underscores the dynamic nature of evolution, where species continually adapt and optimize their traits to better suit their environment, often leading to remarkable specializations like those seen in penguins. By examining these adaptations, we gain a deeper appreciation for the intricate balance of evolutionary pressures that shape the diversity of life on Earth.
Body Structure Modifications
When examining the evolutionary trade-offs that have led to penguins' inability to fly, it is crucial to delve into the significant body structure modifications these birds have undergone. Over millions of years, penguins have evolved from flying ancestors to highly specialized aquatic creatures, a transformation that has necessitated profound changes in their anatomy. One of the most striking modifications is the evolution of their wings. While their ancestors had broad, feathered wings ideal for flight, modern penguins possess wings that are more akin to flippers. These streamlined appendages are perfectly suited for propelling through water with great efficiency, allowing penguins to dive to remarkable depths in search of food. However, this adaptation comes at the cost of aerial mobility; the rigid bones and tightly packed feathers of their flippers make them incapable of generating the lift and maneuverability required for flight. Another critical modification is the alteration in their skeletal structure. Penguins have heavier bones compared to flying birds, which helps them maintain buoyancy underwater but adds weight that would be detrimental for flight. Additionally, their keel bone—the breastbone to which flight muscles attach—is much smaller than that of flying birds, reflecting the reduced need for powerful chest muscles. This shift is complemented by changes in their feather structure; penguins have dense, tightly packed feathers that provide insulation and reduce drag in water but would create too much resistance in air. The body shape of penguins has also been optimized for aquatic life. Their streamlined bodies reduce drag as they swim, while their webbed feet act as powerful paddles. This hydrodynamic design contrasts sharply with the more aerodynamic shapes of flying birds, which are typically lighter and more angular to cut through the air with ease. Furthermore, penguins' eyes are adapted for underwater vision, capable of focusing both in and out of water—a trait that is less beneficial for aerial navigation. In summary, the body structure modifications of penguins represent a prime example of evolutionary trade-offs. These changes have enabled them to thrive in their aquatic environment but have come at the expense of their ability to fly. This trade-off underscores the principle that evolution often involves compromises; in this case, penguins have sacrificed flight for unparalleled swimming prowess, illustrating how different environments can drive distinct anatomical adaptations. Understanding these modifications provides valuable insights into why penguins cannot fly and highlights the intricate balance between form and function in evolutionary biology.
Anatomical Limitations
The intricate world of anatomy is replete with fascinating complexities and limitations that shape the capabilities and behaviors of living organisms. When examining the anatomical constraints of various species, it becomes clear that these limitations are not mere hindrances but rather evolutionary adaptations that have been finely tuned over millennia. This article delves into the anatomical limitations that influence the flight capabilities of birds, focusing on three critical aspects: Wing Morphology and Function, Feather Structure and Aerodynamics, and Skeletal System Constraints. By exploring how these anatomical features interact and impose limitations, we gain insight into the delicate balance between form and function. Understanding these limitations not only highlights the remarkable engineering of biological systems but also underscores the evolutionary trade-offs that have shaped the diversity of life on Earth. As we navigate through these supporting ideas, we will see how each anatomical feature contributes to a broader narrative of evolutionary trade-offs, revealing the intricate compromises that underpin the survival and success of species.
Wing Morphology and Function
Wing morphology and function are critical aspects to consider when understanding why penguins are flightless. Unlike the wings of flying birds, which are adapted for aerodynamics and lift, penguin wings have evolved to serve a different primary purpose. The wings of penguins are more akin to flippers, with a rigid structure that allows for powerful propulsion through water rather than air. This adaptation is a result of their aquatic lifestyle, where the ability to swim efficiently and maneuver underwater is paramount. The shape and size of penguin wings are key factors in their swimming performance. The wings are generally shorter and more robust compared to those of flying birds, with a higher bone density that helps reduce buoyancy in the water. The flipper-like shape allows penguins to generate significant force with each stroke, enabling them to achieve high speeds and agility while chasing prey or escaping predators. Additionally, the feathers on penguin wings are tightly packed and have a unique structure that helps to reduce drag in the water, further enhancing their swimming efficiency. Another important aspect of wing morphology in penguins is the skeletal system. The bones in their wings are fused together in a way that provides rigidity and strength, essential for withstanding the forces generated during swimming. This fusion also limits the flexibility needed for flapping and lift generation, making flight impossible. Furthermore, the muscles associated with wing movement in penguins are adapted for powerful contractions rather than the rapid, repetitive motions required for flying. The functional trade-offs between flying and swimming are evident in the anatomical limitations of penguin wings. While their wings excel at propelling them through water, they lack the necessary characteristics to generate lift and sustain flight in the air. This specialization highlights the evolutionary pressures that have shaped penguin anatomy over time, prioritizing survival and success in their aquatic environment over the ability to fly. In essence, the unique morphology and function of penguin wings are a testament to the remarkable adaptability of these birds and the specific ecological niches they occupy.
Feather Structure and Aerodynamics
The structure and aerodynamics of feathers play a crucial role in understanding why penguins are unable to fly. Feathers, the primary component of avian flight, are intricately designed to provide lift, thrust, and control. However, in penguins, these structures have evolved to serve different purposes. A feather consists of a central shaft (rachis) with vanes (barbs) branching off from it, which are further divided into barbules. In flying birds, the barbules have tiny hooks that zip together to form a tight, smooth surface essential for generating lift and reducing drag. In contrast, penguin feathers lack these hooks, resulting in a more open and dense structure that traps air next to the skin, providing excellent insulation in cold water but hindering aerodynamic efficiency. The aerodynamic principles that govern flight are also compromised in penguins. Lift is generated by the shape of the wing and the movement of air over it. Flying birds have long, narrow wings with a curved upper surface and a flat lower surface, creating an area of lower air pressure above the wing and higher pressure below, thus lifting the bird upwards. Penguins, however, have short, rigid wings that are more suited for propelling themselves through water than through air. The wing shape and movement in penguins are adapted for diving and swimming, where they use their wings like flippers to generate thrust and maneuverability underwater. Additionally, the weight and body structure of penguins further limit their ability to fly. Flying requires a significant amount of energy and a lightweight yet strong skeleton, which is achieved through hollow bones and air-filled cavities in flying birds. Penguins have solid bones and a more robust skeleton that supports their diving lifestyle but adds weight that would be detrimental for flight. Their streamlined bodies, while perfect for gliding through water with minimal resistance, do not allow for the necessary lift and control in the air. In summary, the unique structure of penguin feathers and their adapted aerodynamics are key anatomical limitations that prevent them from flying. These adaptations have evolved to optimize their performance in their aquatic environment, making them highly efficient swimmers and divers but incapable of flight. This specialization highlights the remarkable diversity and adaptability of avian anatomy, where different species have evolved to excel in various environments at the expense of other capabilities.
Skeletal System Constraints
The skeletal system of penguins is a prime example of anatomical limitations that preclude them from flight. Unlike birds that are adapted for flight, such as eagles and sparrows, penguins have several skeletal constraints that make flying impossible. One key limitation is the structure of their wings. While they appear wing-like, these flippers are actually modified forelimbs that are highly specialized for swimming and diving rather than generating lift in the air. The bones in these flippers are dense and heavy, which aids in propulsion through water but would be a significant hindrance in the air due to their weight and rigidity. Another critical constraint is the overall body shape and bone density of penguins. Their bodies are streamlined for aquatic efficiency, with a torpedo-shaped torso that minimizes drag in water but does not provide the necessary aerodynamics for flight. Additionally, their bones are denser than those of flying birds, which helps them dive to great depths without collapsing under pressure but makes them too heavy to achieve the lift required for flight. The skeletal system also lacks the necessary air-filled cavities (pneumatization) found in flying birds. These cavities reduce the overall weight of the skeleton, allowing flying birds to achieve the lightness needed for flight. Penguins, on the other hand, have solid bones that contribute to their overall weight and make flight unfeasible. Furthermore, the keel bone (sternum), which is a crucial anchor point for flight muscles in flying birds, is significantly smaller in penguins. This smaller keel bone means there is less surface area for the powerful pectoral muscles necessary for flapping wings to attach, further limiting any potential for flight. Lastly, the arrangement and structure of other skeletal elements such as the pelvis, legs, and tail feathers also play a role in penguins' inability to fly. Their legs are set far back on their bodies, which is ideal for swimming but not conducive to the balance and stability required during takeoff and landing. In summary, the unique adaptations of penguins' skeletal systems are tailored for their aquatic environment rather than an aerial one. These anatomical limitations ensure that while penguins excel in their natural habitat beneath the waves, they are incapable of taking to the skies like their avian counterparts. This specialization highlights the remarkable diversity within the bird family and underscores how different species evolve specific traits to thrive in their respective environments.
Physiological Constraints
Physiological constraints play a crucial role in determining the performance and adaptability of organisms, influencing how they interact with their environment and evolve over time. These constraints are multifaceted, affecting various aspects of an organism's physiology. For instance, muscle composition and strength significantly impact an organism's ability to perform physical tasks, such as locomotion and predation. The respiratory system's capabilities also set limits on endurance and high-intensity activities by regulating oxygen intake and carbon dioxide expulsion. Additionally, weight and aerodynamic resistance can hinder or enhance movement efficiency, particularly in species that rely on flight or rapid terrestrial locomotion. Understanding these physiological constraints is essential because they often lead to evolutionary trade-offs, where improvements in one area may come at the expense of another. This delicate balance shapes the evolutionary trajectory of species, driving adaptations that optimize survival and reproductive success within specific ecological niches. By exploring these constraints, we can gain deeper insights into the intricate relationships between physiology, environment, and evolution.
Muscle Composition and Strength
When examining the physiological constraints that prevent penguins from flying, it is crucial to delve into the intricacies of muscle composition and strength. Penguins, unlike their flying avian counterparts, have evolved to optimize their muscular structure for swimming and diving rather than flight. The primary distinction lies in the type and distribution of muscle fibers. Flying birds typically possess a high proportion of fast-twitch (FT) muscle fibers, which are specialized for explosive, high-intensity contractions necessary for the rapid wing movements required in flight. In contrast, penguins predominantly have slow-twitch (ST) muscle fibers, which are more efficient for sustained, low-intensity activities such as swimming and diving. The ST fibers in penguins are rich in myoglobin and mitochondria, allowing them to generate energy through aerobic respiration. This adaptation enables penguins to maintain prolonged periods of activity underwater without succumbing to fatigue. Additionally, their muscles are densely packed with myofibrils, enhancing their endurance capabilities. However, this specialization comes at the cost of reduced power output; penguins lack the explosive strength needed to generate the lift and thrust required for flight. Another critical aspect is the arrangement and size of muscles relative to body weight. Flying birds have large pectoral muscles that account for a significant portion of their body weight, facilitating the powerful wing movements necessary for lift-off and sustained flight. Penguins, on the other hand, have relatively smaller pectoral muscles compared to their body size, which are better suited for propelling themselves through water rather than air. Furthermore, the skeletal system of penguins also plays a role in their muscle composition and strength. Their bones are denser and more rigid, which aids in reducing buoyancy and enhancing diving efficiency but makes them less suitable for the lightweight, hollow bones found in flying birds. This structural difference further constrains their ability to generate the necessary lift and thrust for flight. In summary, the unique muscle composition and strength profile of penguins are tailored for aquatic rather than aerial environments. Their reliance on ST fibers, dense muscle packing, and specialized skeletal structure makes them highly efficient swimmers but incapable of flight. This physiological adaptation underscores one of the key reasons why penguins cannot fly despite their evolutionary kinship with flying birds.