Why Does Electronegativity Decrease Down A Group
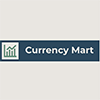
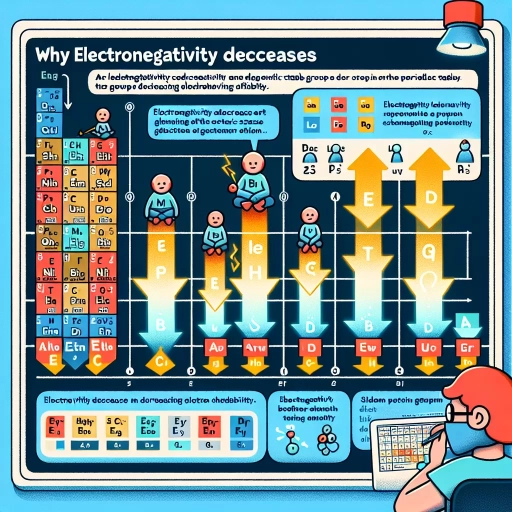
Electronegativity, a fundamental concept in chemistry, measures an atom's ability to attract electrons in a covalent bond. One of the key trends observed in the periodic table is the decrease in electronegativity as you move down a group. This phenomenon is not merely a simple observation but is rooted in several underlying factors. To understand why electronegativity decreases down a group, it is crucial to delve into three primary aspects: the nature of electronegativity itself, the impact of atomic size on this property, and the role of shielding and effective nuclear charge. By examining these elements, we can gain a comprehensive insight into the mechanisms driving this trend. First, we must **Understand Electronegativity and Its Trends**, which sets the foundation for understanding how atomic properties influence electronegativity. This foundational knowledge will then be expanded upon by exploring **Atomic Size and Its Impact on Electronegativity**, as well as **Shielding and Effective Nuclear Charge**, providing a complete picture of why electronegativity diminishes as you descend a group in the periodic table. Let us begin by **Understanding Electronegativity and Its Trends** to lay the groundwork for this detailed analysis.
Understanding Electronegativity and Its Trends
Electronegativity, a fundamental concept in chemistry, plays a crucial role in understanding the behavior of atoms within molecules. It is the measure of an atom's ability to attract and hold onto electrons in a covalent bond. To delve into the intricacies of electronegativity, it is essential to first grasp its definition and how it is measured. This involves understanding the various scales and methods used to quantify this property, which will be explored in the section on **Definition and Measurement of Electronegativity**. Additionally, recognizing the general trends of electronegativity across the periodic table is vital, as these patterns help predict chemical properties and reactivity, a topic that will be covered in **General Trends in the Periodic Table**. Finally, appreciating the importance of electronegativity in chemistry reveals its impact on molecular structure, polarity, and overall chemical behavior, which will be discussed in **Importance of Electronegativity in Chemistry**. By examining these aspects, we can gain a comprehensive understanding of electronegativity and its trends, enabling us to better navigate the complexities of chemical interactions and reactions. Understanding Electronegativity and Its Trends is thus a cornerstone of chemical knowledge, essential for both theoretical and practical applications in the field.
Definition and Measurement of Electronegativity
**Definition and Measurement of Electronegativity** Electronegativity is a fundamental concept in chemistry that quantifies the ability of an atom in a molecule to attract shared electrons towards itself. This property is crucial for understanding the nature of chemical bonds and predicting the behavior of molecules. The term was first introduced by Linus Pauling in the 1930s, and since then, it has become a cornerstone in the study of molecular structure and reactivity. The measurement of electronegativity is typically expressed on the Pauling scale, which ranges from approximately 0.7 for cesium (the least electronegative element) to 4.0 for fluorine (the most electronegative element). This scale is relative and based on the difference in electronegativity between atoms in a covalent bond. The Pauling scale is derived from bond energies and is calibrated such that the electronegativity of hydrogen is set at 2.2. Other scales, such as the Mulliken-Jaffe scale and the Allred-Rochow scale, also exist but are less commonly used. Electronegativity is influenced by several factors, including atomic number, nuclear charge, and electron shielding. As atomic number increases within a period (left to right across the periodic table), the number of protons in the nucleus increases, which enhances the nuclear charge and thus the atom's ability to attract electrons. Conversely, moving down a group (top to bottom in the periodic table), the distance between the nucleus and valence electrons increases due to additional energy levels, reducing the effective nuclear charge and resulting in lower electronegativity. The measurement of electronegativity can be approached through various methods, including empirical formulas derived from bond dissociation energies and spectroscopic data. For instance, the Mulliken-Jaffe method uses ionization energies and electron affinities to calculate electronegativities. These methods provide a quantitative basis for comparing the electronegativities of different elements, enabling chemists to predict bond polarities, molecular shapes, and reactivity patterns. Understanding electronegativity is essential for explaining trends in chemical properties and behaviors. For example, it helps explain why certain compounds are more reactive or stable than others. The decrease in electronegativity down a group can be attributed to the increased distance between the nucleus and valence electrons, leading to weaker attraction forces. This trend is critical for understanding various chemical phenomena, such as the reactivity of elements and the formation of ions. By grasping the concept and measurement of electronegativity, chemists can better interpret and predict the chemical behavior of elements and compounds, making it a vital tool in both theoretical and applied chemistry.
General Trends in the Periodic Table
General trends in the periodic table are fundamental to understanding various chemical properties, including electronegativity. The periodic table is arranged in a way that elements with similar properties recur at regular intervals, allowing for the identification of trends. One of the most significant trends is the variation in atomic radius, which generally increases down a group and decreases across a period. This trend is crucial because it directly influences other properties such as electronegativity, ionization energy, and electron affinity. Electronegativity, a measure of an atom's ability to attract electrons in a covalent bond, follows a specific trend. It decreases down a group due to the increase in atomic radius. As you move down a group, the outermost electrons are farther away from the nucleus, reducing the effective nuclear charge and thus the atom's ability to attract electrons. Conversely, electronegativity increases across a period because the atomic radius decreases, bringing the outermost electrons closer to the nucleus and increasing the effective nuclear charge. Another important trend is the variation in ionization energy, which is the energy required to remove an electron from an atom. Ionization energy generally decreases down a group and increases across a period. This trend is inversely related to atomic radius; larger atoms have lower ionization energies because their outer electrons are easier to remove due to being farther from the nucleus. Electron affinity, the energy change when an electron is added to an atom, also exhibits specific trends. It tends to decrease down a group due to the increased atomic radius, making it less favorable for an atom to accept an additional electron. Across a period, electron affinity generally increases as the atomic radius decreases, making it more favorable for an atom to accept an electron. Understanding these trends is essential for predicting chemical behavior and reactivity. For instance, knowing that electronegativity decreases down a group helps explain why certain elements form ions more readily than others. Similarly, recognizing the trend in ionization energy can help predict which elements are more likely to lose or gain electrons in chemical reactions. In summary, the periodic table's arrangement allows for the identification of clear trends in atomic radius, electronegativity, ionization energy, and electron affinity. These trends are interconnected and provide a framework for understanding various chemical properties and behaviors. By recognizing these patterns, chemists can better predict how elements will react and interact with each other, which is vital for both theoretical and practical applications in chemistry.
Importance of Electronegativity in Chemistry
Electronegativity, a fundamental concept in chemistry, plays a crucial role in understanding the behavior of atoms within molecules. It is defined as the ability of an atom to attract and hold onto electrons in a covalent bond. This property is essential for predicting the polarity of bonds, the reactivity of elements, and the overall stability of molecules. When atoms with different electronegativities form a bond, the atom with higher electronegativity pulls the shared electrons closer to itself, creating a partial positive charge on the other atom. This polarity can significantly influence chemical reactions and the physical properties of compounds. For instance, electronegativity helps explain why certain molecules are polar while others are nonpolar. In water (H₂O), oxygen has a higher electronegativity than hydrogen, resulting in a polar molecule with a partial negative charge on oxygen and partial positive charges on hydrogen atoms. This polarity is crucial for water's role as a solvent and its ability to dissolve a wide range of substances. Similarly, in carbon dioxide (CO₂), the difference in electronegativity between carbon and oxygen leads to polar bonds, which contribute to its linear molecular geometry and high reactivity. Understanding electronegativity trends is also vital for predicting chemical reactivity. Elements with high electronegativity tend to form anions more easily because they can attract electrons strongly. Conversely, elements with low electronegativity are more likely to lose electrons and form cations. This knowledge is indispensable in organic chemistry, where the formation of bonds and the stability of intermediates are often determined by electronegativity differences. Moreover, electronegativity affects the acid-base properties of molecules. In hydrogen fluoride (HF), fluorine's high electronegativity makes it a strong acid because it can readily release a proton (H⁺). In contrast, hydrogen iodide (HI) is a weak acid due to iodine's lower electronegativity, which results in a weaker bond between hydrogen and iodine. The decrease in electronegativity down a group in the periodic table is another critical aspect. As you move down a group, the atomic radius increases, and the outermost electrons are farther away from the nucleus. This increased distance reduces the effective nuclear charge felt by these electrons, leading to lower electronegativity values. For example, fluorine has the highest electronegativity in group 17, while iodine has the lowest. This trend explains why fluorine compounds are generally more reactive and stable than iodine compounds. In summary, electronegativity is a cornerstone of chemical understanding, enabling chemists to predict bond polarity, reactivity, and molecular stability. Its trends down groups in the periodic table provide insights into why certain elements behave differently under various conditions. By grasping these concepts, chemists can better design experiments, synthesize new compounds, and understand complex chemical phenomena.
Atomic Size and Its Impact on Electronegativity
Atomic size, a fundamental concept in chemistry, plays a crucial role in understanding the behavior of elements, particularly in relation to their electronegativity. Electronegativity, the measure of an atom's ability to attract electrons in a covalent bond, is influenced by several factors, including atomic size. This article delves into the intricate relationship between atomic size and electronegativity, exploring three key aspects: the increase in atomic radius down a group, the effect of atomic size on electron attraction, and the shielding effect by inner electrons. By examining these concepts, we can gain a deeper understanding of how atomic size impacts electronegativity and its trends. As we navigate through these supporting ideas, we will transition seamlessly into a comprehensive understanding of electronegativity and its trends, providing a robust framework for appreciating the complex interplay between atomic structure and chemical properties. This exploration will not only enhance our knowledge of atomic interactions but also shed light on the underlying principles that govern chemical bonding and reactivity.
Increase in Atomic Radius Down a Group
As we descend a group in the periodic table, a significant trend emerges: the atomic radius increases. This phenomenon is crucial for understanding various chemical properties, including electronegativity. The increase in atomic radius down a group is primarily due to the addition of new energy levels or electron shells. Each subsequent element in a group adds an extra principal energy level, which results in the outermost electrons being farther away from the nucleus. This increased distance reduces the effective nuclear charge experienced by these outer electrons, leading to a weaker attraction between the nucleus and the outer electrons. Consequently, as the atomic radius increases, the electrons are less tightly bound to the nucleus. This reduced binding energy makes it easier for atoms to lose or gain electrons, influencing their reactivity and chemical behavior. For instance, alkali metals in Group 1 exhibit this trend prominently; as you move down from lithium to cesium, the atomic radius increases significantly, making cesium more reactive due to its larger size and lower ionization energy. The impact of this trend on electronegativity is profound. Electronegativity, a measure of an atom's ability to attract electrons in a covalent bond, decreases down a group because the increased atomic radius results in a lower effective nuclear charge on the outer electrons. With a weaker pull from the nucleus, these atoms are less capable of attracting shared electrons towards themselves. This decrease in electronegativity down a group explains why elements at the bottom of a group tend to form weaker bonds and are generally more reactive compared to their counterparts at the top. Understanding this relationship between atomic radius and electronegativity is essential for predicting chemical behavior and reactivity patterns. For example, in Group 17 (the halogens), fluorine has the highest electronegativity due to its small atomic radius and strong nuclear pull on its outer electrons. In contrast, iodine, with its larger atomic radius and lower effective nuclear charge, has significantly lower electronegativity. This difference in electronegativity influences their ability to form bonds and participate in chemical reactions. In summary, the increase in atomic radius down a group is a fundamental principle that underpins many chemical properties. It leads to a decrease in electronegativity, which in turn affects how atoms interact with each other and form bonds. Recognizing this trend is vital for comprehending various aspects of chemistry and predicting the behavior of elements across different groups in the periodic table.