Why Is The Moon Rusting
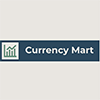
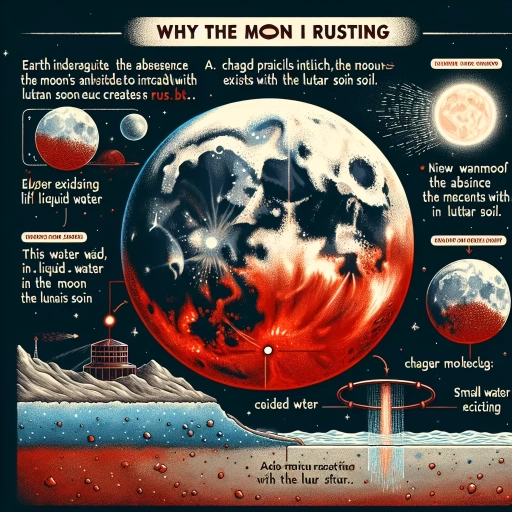
The moon, often perceived as a pristine and unchanging celestial body, is undergoing a surprising transformation: it is rusting. This phenomenon, though seemingly counterintuitive given the moon's lack of atmosphere and liquid water, has sparked significant interest among scientists. The rusting of the moon is not just a curiosity but a complex process influenced by various factors. To understand this phenomenon, it is crucial to delve into the introduction to lunar rusting, where we explore the foundational aspects of how and why this occurs. We will also examine the chemical and environmental factors that contribute to lunar rusting, including the role of solar winds and the presence of water ice. Finally, we will discuss the scientific implications of this discovery and the future research directions that aim to uncover more about this enigmatic process. By understanding these elements, we can gain a deeper insight into the moon's evolving surface and its broader implications for space exploration. Let us begin with an introduction to lunar rusting to set the stage for this fascinating exploration.
Introduction to Lunar Rusting
The phenomenon of lunar rusting, though seemingly paradoxical given the Moon's lack of atmosphere, has garnered significant attention in recent years. This intriguing topic is deeply rooted in the historical context of lunar exploration, which has been a cornerstone of space research since the mid-20th century. As we delve into the history, we uncover the initial observations and theories that first hinted at the presence of rust on the Moon's surface. These early findings were pivotal in shaping our understanding of lunar geology and the Moon's interaction with its environment. The significance of understanding lunar rusting extends beyond mere curiosity; it holds crucial implications for future lunar missions and the potential for resource utilization on the Moon. By exploring these facets—historical context, initial observations, and the significance of understanding lunar rusting—we can gain a comprehensive insight into this fascinating phenomenon. This article aims to provide an in-depth introduction to lunar rusting, shedding light on its complexities and importance in the broader landscape of space exploration. **Introduction to Lunar Rusting**
Historical Context of Lunar Exploration
The historical context of lunar exploration is a rich and fascinating narrative that sets the stage for understanding the phenomenon of lunar rusting. The journey to the Moon began in earnest during the Cold War era, with the Soviet Union launching the first successful lunar mission, Luna 2, in 1959. This was followed by a series of Soviet and American missions aimed at exploring the Moon's surface. One of the most pivotal moments came in 1969 when NASA's Apollo 11 mission successfully landed astronauts Neil Armstrong and Buzz Aldrin on the lunar surface, marking a historic milestone in space exploration. Subsequent Apollo missions continued to expand our knowledge of the Moon, with each mission contributing valuable data and samples. The Apollo 15, 16, and 17 missions, for example, included the deployment of lunar rovers that allowed astronauts to traverse greater distances and conduct more extensive scientific experiments. These missions not only provided insights into the Moon's geology but also laid the groundwork for future lunar research. In the decades following the Apollo era, lunar exploration experienced a lull, but interest was rekindled in the late 20th and early 21st centuries. Missions such as NASA's Lunar Prospector (1998-1999) and the European Space Agency's SMART-1 (2003-2006) renewed scientific interest in the Moon. More recently, China's Chang'e program has made significant strides, including the successful landing of the Chang'e 4 mission on the far side of the Moon in 2019. This cumulative effort has led to a deeper understanding of the Moon's composition, atmosphere, and environmental conditions. However, it also revealed unexpected phenomena such as lunar rusting—a process where iron-rich materials on the lunar surface react with oxygen to form rust. This discovery challenges our previous assumptions about the Moon's airless environment and highlights the complexity of lunar chemistry. Understanding lunar rusting requires a comprehensive look at how these historical missions have contributed to our current knowledge. The samples brought back by Apollo astronauts, combined with data from more recent robotic missions, provide critical insights into the chemical and physical processes occurring on the Moon. As we continue to explore and study our closest celestial neighbor, unraveling mysteries like lunar rusting will be essential for future lunar missions and potential human settlements. The historical context of lunar exploration thus serves as a foundation for ongoing research, underscoring the importance of continued scientific inquiry into the Moon's enigmatic surface.
Initial Observations and Theories
Initial observations and theories regarding lunar rusting have been a subject of intrigue and scientific investigation. The phenomenon was first noted during the Apollo missions, where astronauts observed reddish hues on the lunar surface, particularly in areas with high concentrations of iron-rich minerals. These early observations sparked a series of hypotheses aimed at understanding the underlying mechanisms. One of the initial theories suggested that lunar rusting could be the result of oxidation reactions involving iron and oxygen, similar to those that cause rust on Earth. However, this theory faced significant challenges due to the Moon's extremely low atmospheric pressure and lack of liquid water, both of which are crucial for typical rusting processes. Subsequent research proposed alternative explanations, including the role of solar wind and meteorite impacts. Scientists hypothesized that the solar wind, rich in oxygen ions, could interact with the lunar surface to facilitate oxidation reactions. Additionally, meteorite impacts were considered as potential sources of oxygen and other reactive species that might contribute to rusting. These theories were supported by laboratory experiments simulating lunar conditions, which showed that iron oxides could form under such environments. Further investigation revealed that lunar regolith, the loose, fragmented rock that covers the Moon's surface, plays a critical role in the rusting process. The regolith is highly reactive due to its exposure to cosmic radiation and solar wind, leading to the formation of reactive oxygen species. These species can then react with iron-bearing minerals to produce iron oxides, resulting in the characteristic reddish color observed on the Moon's surface. The integration of these findings has led to a more comprehensive understanding of lunar rusting. It is now recognized as a complex process influenced by multiple factors, including solar wind, meteorite impacts, and the chemical reactivity of lunar regolith. This understanding not only sheds light on the Moon's surface chemistry but also has broader implications for future lunar missions and the potential for resource utilization on the Moon. As research continues to refine our knowledge of lunar rusting, it underscores the dynamic and chemically active nature of the Moon's surface, challenging traditional views of a static and inert environment.
Significance of Understanding Lunar Rusting
Understanding lunar rusting is a pivotal aspect of space exploration and planetary science, offering profound insights into the Moon's surface chemistry, geological history, and potential implications for future lunar missions. The phenomenon of lunar rusting, where iron-rich minerals react with oxygen to form iron oxides, is not as straightforward as its terrestrial counterpart due to the Moon's unique environment. Unlike Earth, where rusting is driven by moisture and oxygen in the atmosphere, the Moon's surface lacks liquid water but is exposed to solar winds and meteoritic impacts that introduce oxygen and other reactive species. This process can alter the physical and chemical properties of lunar regolith, affecting its reflectivity, thermal conductivity, and mechanical strength. Comprehending lunar rusting is crucial for several reasons. Firstly, it helps scientists understand the Moon's geological evolution and how its surface has been shaped over billions of years. By analyzing the extent and patterns of rusting, researchers can infer details about past environmental conditions, including the presence of water ice or other volatile compounds. Secondly, understanding lunar rusting is essential for planning and executing future lunar missions. For instance, knowing how rusting affects the mechanical properties of regolith can inform the design of landing sites and habitats, ensuring that they are stable and safe for both robotic and human exploration. Additionally, the study of lunar rusting has broader implications for understanding similar processes on other celestial bodies, such as Mars, where iron oxides play a significant role in shaping the planet's surface. Moreover, the study of lunar rusting intersects with technological advancements in materials science. By understanding how iron-rich materials degrade in extraterrestrial environments, scientists can develop more resilient materials for spacecraft and equipment designed for long-term exposure to space conditions. This knowledge can also contribute to the development of sustainable technologies for lunar resource utilization, such as extracting oxygen from lunar regolith—a critical component for life support systems and propulsion in future missions. In summary, the significance of understanding lunar rusting lies in its multifaceted impact on our comprehension of lunar geology, the planning of future space missions, and the advancement of materials science. As we continue to explore and utilize the Moon's resources, a deep understanding of this phenomenon will be indispensable for ensuring the success and sustainability of our endeavors in space.
Chemical and Environmental Factors
The exploration of the Moon has unveiled a complex interplay of chemical and environmental factors that significantly influence its surface and subsurface conditions. As we delve into the intricacies of lunar chemistry, three key aspects emerge as pivotal: the presence of water and oxygen, the role of solar wind and cosmic rays, and the impact of lunar regolith composition. The presence of water and oxygen on the Moon, though in trace amounts, has profound implications for potential human habitation and resource utilization. Meanwhile, solar wind and cosmic rays continuously bombard the lunar surface, altering its chemical landscape and posing challenges for both robotic and human missions. Additionally, the composition of lunar regolith plays a crucial role in understanding the Moon's geological history and its potential for supporting future technological endeavors. These factors collectively set the stage for a deeper exploration of a phenomenon that could have significant implications for long-term lunar missions: lunar rusting. In this article, we will transition into an in-depth examination of **Introduction to Lunar Rusting**, highlighting how these chemical and environmental factors contribute to this critical issue.
Presence of Water and Oxygen on the Moon
The presence of water and oxygen on the Moon is a crucial aspect to consider when discussing the phenomenon of lunar rusting, a topic that falls under the broader category of chemical and environmental factors. Historically, the Moon was thought to be a completely dry and airless body, devoid of any significant water or oxygen reserves. However, recent discoveries have challenged this long-held belief. NASA's Lunar CRater Observation and Sensing Satellite (LCROSS) mission in 2009 revealed the presence of water ice in the permanently shadowed craters near the lunar poles. This finding was further corroborated by subsequent missions and observations, including those from India's Chandrayaan-1 and NASA's Lunar Reconnaissance Orbiter. The existence of water on the Moon has significant implications for understanding lunar chemistry and potential environmental interactions. Water molecules can dissociate into hydrogen and oxygen under solar radiation, a process known as photodissociation. This process contributes to the lunar exosphere, which, although very thin, contains trace amounts of oxygen. The presence of oxygen, albeit in small quantities, is critical because it can react with metallic components on the lunar surface, such as iron, to form oxides—essentially rust. Moreover, the interaction between lunar regolith (soil) and solar wind also plays a role in the chemical environment of the Moon. Solar wind, rich in hydrogen ions, can react with lunar silicates to produce water molecules, further enriching the lunar surface with hydrogen and oxygen. These chemical reactions are pivotal in understanding how rusting could occur on the Moon's surface, especially in areas where metallic debris from spacecraft or other human activities might be present. In addition to these chemical processes, the environmental conditions on the Moon—such as extreme temperature fluctuations and radiation exposure—also influence the stability and reactivity of water and oxygen. For instance, the lunar surface experiences temperatures ranging from extremely cold to very hot, which can affect the volatility and reactivity of water molecules and their by-products. In summary, the presence of water and oxygen on the Moon, albeit in trace amounts, sets the stage for understanding how rusting could occur in this seemingly inhospitable environment. The interplay between solar radiation, lunar regolith, and solar wind creates a complex chemical landscape where reactions involving water and oxygen can lead to the formation of rust. This insight is crucial for future lunar missions and the long-term sustainability of human presence on the Moon, as it highlights the need to consider and mitigate potential chemical interactions that could affect both natural and artificial materials on the lunar surface.
Role of Solar Wind and Cosmic Rays
The role of solar wind and cosmic rays in the lunar environment is a critical factor in understanding why the Moon appears to be "rusting." Solar wind, composed primarily of charged particles such as protons and electrons, emanates from the Sun and interacts with the lunar surface. These particles can penetrate the Moon's surface, causing chemical reactions that alter its composition. For instance, when solar wind protons collide with lunar regolith, they can react with oxygen atoms to form water (H₂O) and hydroxyl (OH) molecules. This process, known as solar wind implantation, contributes to the hydration of lunar minerals, which in turn can lead to the formation of rust-like compounds. Cosmic rays, high-energy particles originating from outside the solar system, also play a significant role. These particles are capable of penetrating deeper into the lunar regolith than solar wind particles, inducing further chemical changes. Cosmic rays can ionize and break down molecules, leading to the formation of reactive species that interact with metal oxides present in lunar soil. This interaction can result in the oxidation of iron and other metals, contributing to the rusting phenomenon observed on the Moon. The combined effects of solar wind and cosmic rays create a dynamic and chemically active environment on the lunar surface. These processes not only influence the chemical composition but also affect the physical properties of lunar materials. For example, the continuous bombardment by solar wind and cosmic rays can lead to the formation of defects in mineral structures, enhancing their reactivity and susceptibility to further chemical transformations. Moreover, these environmental factors are exacerbated by the Moon's lack of a protective atmosphere and magnetic field, which would otherwise shield it from such particle fluxes. As a result, the lunar surface is exposed to a constant barrage of energetic particles, making it an ideal laboratory for studying the effects of space weathering on planetary bodies. In summary, the interaction between solar wind and cosmic rays with the lunar surface is a key driver behind the observed rusting of the Moon. These processes highlight the complex interplay between extraterrestrial radiation and planetary chemistry, underscoring the importance of considering these factors when interpreting geological phenomena on celestial bodies like the Moon.
Impact of Lunar Regolith Composition
The composition of lunar regolith, the loose, fragmented rock that covers the Moon's surface, plays a crucial role in understanding the chemical and environmental factors contributing to the phenomenon often referred to as "Moon rusting." Lunar regolith is primarily composed of silicate minerals, metals, and other inorganic compounds, which are the result of meteorite impacts, solar wind bombardment, and thermal processes. These components interact with the harsh lunar environment in ways that significantly influence the Moon's surface chemistry. For instance, the presence of iron and titanium oxides in the regolith can react with oxygen and water molecules introduced by solar wind or cometary impacts, leading to oxidation reactions. These reactions can produce rust-like compounds, such as iron oxides and hydroxides, which are responsible for the reddish hue observed in some lunar samples. Additionally, the fine-grained nature of lunar regolith enhances its reactivity due to its high surface area, facilitating chemical interactions that would be less pronounced in coarser materials. The absence of a protective atmosphere on the Moon means that these reactions occur without the moderating influence of air, allowing them to proceed more rapidly and extensively. Furthermore, the extreme temperature fluctuations between day and night on the Moon's surface can accelerate these chemical processes by repeatedly expanding and contracting the regolith particles, thereby increasing their mechanical stress and reactivity. Understanding the impact of lunar regolith composition is essential for interpreting the Moon's surface chemistry and predicting how future human activities might alter or interact with this environment. This knowledge also has broader implications for planetary science, as it helps scientists model similar processes on other airless bodies in the solar system, providing insights into their geological and chemical evolution. In summary, the composition of lunar regolith is a key factor in the complex interplay of chemical and environmental processes that shape the Moon's surface, contributing to phenomena like "Moon rusting" and informing our broader understanding of planetary chemistry.
Scientific Implications and Future Research
The scientific implications and future research in space exploration are pivotal as we continue to push the boundaries of human knowledge and technological capabilities. One of the critical areas of focus is the Potential for In-Situ Resource Utilization (ISRU), which promises to revolutionize how we sustain long-term missions by leveraging resources found in space. Another significant aspect is the Impact on Future Lunar Missions and Habitats, where understanding the lunar environment will be crucial for establishing sustainable human presence on the Moon. Additionally, Advancements in Materials Science and Technology are driving innovation, enabling the development of more resilient and efficient materials for space applications. These interconnected themes highlight the multifaceted nature of space research and its potential to transform our understanding of the cosmos. As we delve into these topics, it becomes clear that each area of study has profound implications for our future in space. This article will explore these scientific implications in depth, ultimately transitioning to a detailed examination of a specific challenge: Introduction to Lunar Rusting.
Potential for In-Situ Resource Utilization (ISRU)
In-Situ Resource Utilization (ISRU) holds immense potential for future lunar missions, significantly enhancing their sustainability and reducing reliance on Earth-based supplies. By leveraging resources available on the Moon, such as water ice, lunar regolith, and atmospheric gases, ISRU can provide essential materials for life support, propulsion, and construction. For instance, water extracted from lunar ice can be split into hydrogen and oxygen through electrolysis, yielding oxygen for breathing and hydrogen for fueling spacecraft. This capability not only extends mission durations but also enables the creation of fuel depots that could support deeper space exploration. Additionally, lunar regolith can be processed to produce oxygen, metals, and other valuable resources, facilitating the construction of habitats, solar panels, and other infrastructure necessary for establishing a permanent human presence on the Moon. The scientific implications of ISRU are profound; it could revolutionize our approach to space exploration by making long-term missions more feasible and cost-effective. However, further research is needed to overcome technical challenges such as efficient extraction methods, resource processing technologies, and the development of robust systems capable of operating in the harsh lunar environment. Investigating these aspects will be crucial for unlocking the full potential of ISRU and paving the way for a sustainable human presence in space. As we continue to explore the Moon and beyond, the integration of ISRU into mission planning will be a key factor in achieving our goals of expanding human knowledge and presence in the cosmos.
Impact on Future Lunar Missions and Habitats
The impact on future lunar missions and habitats due to the moon's rusting phenomenon is multifaceted and significant. As scientists continue to unravel the mysteries behind the lunar regolith's reddish hue, several key implications emerge for both short-term and long-term space exploration endeavors. Firstly, understanding the chemical processes that lead to rusting on the moon is crucial for designing durable materials and equipment for lunar missions. The presence of water ice in permanently shadowed craters, which can react with iron-rich minerals to form rust, highlights the need for robust protective coatings and materials that can withstand these corrosive conditions. This knowledge will be essential for the longevity of lunar landers, rovers, and habitats, ensuring they remain functional over extended periods. Moreover, the discovery of rust on the moon raises important questions about the availability of resources for future human settlements. If lunar regolith can react with water to produce rust, it suggests that there may be accessible sources of water and oxygen, which are vital for sustaining life and propulsion systems. However, this also means that any infrastructure built on the moon must be designed with these reactive processes in mind to prevent structural degradation. For instance, habitats constructed from lunar materials could potentially be engineered to harness these reactions beneficially, such as extracting oxygen from the regolith or using it as a resource for life support systems. Additionally, the study of lunar rusting has broader scientific implications that extend beyond immediate practical applications. It provides a unique window into the moon's geological history and its interaction with the solar wind and meteoritic impacts. By analyzing the distribution and intensity of rusting across different regions of the moon, scientists can gain insights into past environmental conditions and the moon's evolution over billions of years. This research can also inform our understanding of similar processes on other celestial bodies, such as Mars, where rusting could play a significant role in shaping surface chemistry and potential habitability. In terms of future research directions, the phenomenon of lunar rusting opens up several avenues for investigation. One area involves detailed spectroscopic studies to map the distribution of rust across the lunar surface accurately. Another involves laboratory experiments to replicate the conditions under which rust forms on the moon, allowing for a deeper understanding of the underlying chemical mechanisms. Furthermore, integrating this knowledge into mission planning will be critical; for example, selecting landing sites that minimize exposure to corrosive environments or developing technologies that can mitigate these effects. Ultimately, the impact of lunar rusting on future missions and habitats underscores the importance of interdisciplinary research in space exploration. By combining insights from geology, chemistry, materials science, and engineering, we can better prepare for the challenges and opportunities presented by this phenomenon. As we move forward with plans to return humans to the moon and establish sustainable presence there, understanding and addressing the implications of lunar rusting will be essential for ensuring the success and longevity of these endeavors.
Advancements in Materials Science and Technology
Advancements in materials science and technology have significantly impacted our understanding and interaction with various environments, including the lunar surface. One of the most intriguing recent discoveries is the phenomenon of "rusting" on the Moon, which challenges traditional notions about the Moon's airless and supposedly inert environment. This observation has sparked a flurry of scientific interest and research, highlighting the importance of advanced materials analysis. At the forefront of these advancements are sophisticated spectroscopic techniques and high-resolution imaging technologies. These tools allow scientists to detect and analyze subtle changes in material properties at the molecular level, even in extreme environments like those found on the Moon. For instance, the use of X-ray fluorescence (XRF) and laser-induced breakdown spectroscopy (LIBS) has enabled researchers to identify iron oxide compounds—essentially rust—on lunar samples. These findings suggest that water molecules, possibly introduced by comets or solar winds, can react with iron in lunar regolith to form rust, a process previously thought to be impossible without oxygen. The implications of these discoveries extend beyond lunar research; they also have profound effects on our understanding of planetary chemistry and the potential for life on other celestial bodies. For example, if rust can form on the Moon, it may indicate similar processes occurring on Mars or other planets with iron-rich soils. This could provide insights into the presence of water and potential biosignatures, making these advancements crucial for future astrobiological research. Moreover, the study of lunar rust has driven innovations in materials engineering. Understanding how materials degrade or transform under extreme conditions helps in developing more resilient and durable materials for space exploration. For instance, designing spacecraft components that can withstand the harsh conditions of space, including radiation and extreme temperatures, becomes more feasible with insights gained from lunar material studies. Future research in this area is likely to focus on detailed chemical analyses of lunar samples and in-situ experiments to better understand the mechanisms behind lunar rust formation. Advanced computational models will also play a critical role in simulating these processes and predicting how materials might behave in various extraterrestrial environments. Additionally, interdisciplinary collaborations between materials scientists, planetary geologists, and astrobiologists will be essential for unraveling the full scientific implications of these findings and their broader significance for space exploration and the search for life beyond Earth. As we continue to push the boundaries of materials science and technology, we open new avenues for understanding our universe and its many mysteries.