Why Are Valence Electrons Important
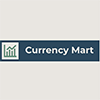
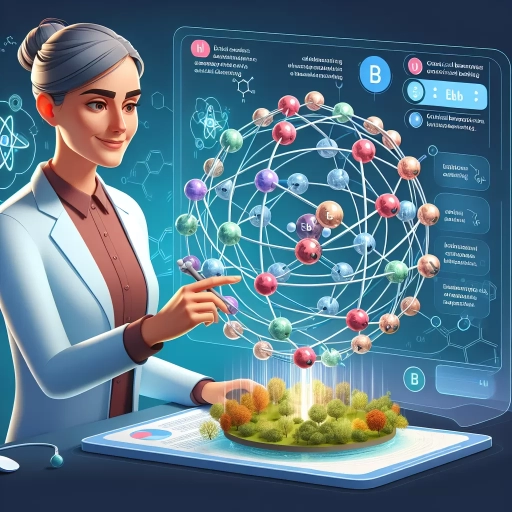
Valence electrons are a fundamental concept in chemistry, playing a crucial role in understanding the behavior and properties of elements. These outermost electrons determine how atoms interact with each other, forming the basis of chemical bonding and influencing the chemical properties of substances. In this article, we will delve into the importance of valence electrons by first exploring the basics of what they are and how they are structured. We will then examine their significance in chemical bonding, highlighting how these electrons facilitate the formation of various types of bonds that hold molecules together. Finally, we will discuss how valence electrons impact the chemical properties of elements, affecting reactivity, conductivity, and other essential characteristics. By grasping these concepts, readers will gain a comprehensive understanding of why valence electrons are indispensable in chemistry. To begin, let's start with the foundational knowledge: **Understanding Valence Electrons: The Basics**.
Understanding Valence Electrons: The Basics
Understanding valence electrons is a fundamental concept in chemistry that underpins the behavior and properties of atoms. These electrons, located in the outermost shell of an atom, play a crucial role in determining how atoms interact with each other to form molecules. To grasp the basics of valence electrons, it is essential to delve into their definition and role within the atomic structure. This involves understanding how valence electrons influence chemical reactivity and bonding. Additionally, knowing their location and configuration within the atom's energy levels is vital for predicting chemical behavior. Finally, comparing valence electrons with core electrons highlights their unique characteristics and importance in chemical processes. By exploring these aspects, we can gain a comprehensive understanding of valence electrons and their significance in chemistry. In this article, we will explore these key concepts to provide a thorough introduction to **Understanding Valence Electrons: The Basics**.
Definition and Role in Atoms
Valence electrons are a fundamental concept in chemistry, playing a crucial role in the structure and behavior of atoms. **Definition**: Valence electrons are the electrons in the outermost shell of an atom, which is the valence shell. These electrons are involved in chemical bonding and determine the chemical properties of an element. The number of valence electrons an atom has is a key factor in its reactivity and how it interacts with other atoms. **Role in Atoms**: The valence shell is the outermost energy level of an atom, and it is here that valence electrons reside. These electrons are highly influential because they participate in forming chemical bonds with other atoms. The arrangement and number of valence electrons dictate whether an atom will form ionic, covalent, or metallic bonds. For instance, atoms with a full outer shell (like noble gases) are chemically inert because their valence shell is complete, while atoms with partially filled outer shells (like metals and nonmetals) are more reactive as they seek to achieve a stable configuration by gaining or losing electrons. The role of valence electrons extends beyond just bonding; they also influence the physical properties of elements. For example, metals typically have one or two valence electrons, which are easily lost to form positive ions (cations), contributing to their high electrical conductivity and malleability. On the other hand, nonmetals have more valence electrons and tend to gain electrons to form negative ions (anions), leading to different physical characteristics such as brittleness and lower conductivity. Understanding valence electrons is essential for predicting how elements will react in various chemical reactions. The octet rule, which states that atoms tend to gain, lose, or share electrons to achieve a full outer shell with eight electrons (except for hydrogen which seeks two), is a direct consequence of valence electron behavior. This principle helps chemists predict the types of compounds that can form and the stability of those compounds. In summary, valence electrons are pivotal in defining the chemical identity and reactivity of an element. Their presence in the outermost shell makes them crucial for forming bonds and determining physical properties. Grasping the concept of valence electrons provides a foundational understanding of atomic behavior, enabling chemists to predict and explain a wide range of chemical phenomena. This knowledge is indispensable for understanding why valence electrons are important in chemistry and how they shape the world around us.
Location and Configuration
**Location and Configuration** Understanding the location and configuration of valence electrons is crucial for grasping their significance in chemistry. Valence electrons are the outermost electrons in an atom, residing in the valence shell, which is the outermost energy level. The arrangement of these electrons determines an atom's chemical properties and its ability to form bonds with other atoms. In a neutral atom, the number of valence electrons corresponds to the group number in the periodic table. For instance, elements in Group 1 (alkali metals) have one valence electron, while those in Group 17 (halogens) have seven. This configuration influences how readily an atom can gain or lose electrons to achieve a stable electronic configuration, often mimicking the noble gas configuration. The configuration of valence electrons is described using electron configuration notation, which specifies how electrons are distributed across different energy levels or orbitals. For example, the electron configuration of carbon (atomic number 6) is \(1s^2 2s^2 2p^2\), indicating that its valence shell contains four electrons. This specific arrangement allows carbon to form four covalent bonds, making it a versatile element in organic chemistry. The spatial distribution of valence electrons is also important. According to the valence shell electron pair repulsion (VSEPR) theory, electron pairs in the valence shell repel each other, leading to specific geometries around the central atom. For example, methane (CH₄) has a tetrahedral shape because the four electron pairs around the carbon atom arrange themselves to minimize repulsion. Moreover, the concept of hybridization explains how valence electrons mix to form hybrid orbitals that are better suited for bonding. In the case of carbon, sp³ hybridization results in four equivalent hybrid orbitals that point towards the corners of a tetrahedron, facilitating the formation of strong covalent bonds with hydrogen atoms in methane. In summary, understanding the location and configuration of valence electrons provides insights into an atom's chemical behavior and its capacity to form bonds. This knowledge is fundamental for predicting molecular shapes, reactivity, and stability, making it a cornerstone in understanding valence electrons and their importance in chemistry.
Comparison with Core Electrons
When delving into the importance of valence electrons, it is crucial to understand their distinction from core electrons. Valence electrons, located in the outermost shell of an atom, play a pivotal role in chemical bonding and reactivity. In contrast, core electrons reside in the inner shells and are shielded from the nucleus by the valence electrons. This shielding effect significantly reduces the attractive force between core electrons and the nucleus, making them less influential in chemical interactions. The primary difference between valence and core electrons lies in their energy levels and orbital configurations. Valence electrons occupy higher energy levels and are more accessible for bonding due to their proximity to the outer surface of the atom. This accessibility allows them to participate in various types of chemical bonds, such as covalent, ionic, and metallic bonds, which are fundamental to the formation of molecules and compounds. On the other hand, core electrons are tightly bound to the nucleus and require substantial energy to be removed or excited, making them relatively inert in most chemical reactions. Another key aspect is the stability and reactivity associated with these electron types. Valence electrons determine an atom's reactivity because they are involved in electron transfer or sharing during chemical reactions. Atoms strive to achieve a full outer shell configuration, often mimicking the noble gas configuration, which is highly stable. This drive for stability underpins many chemical processes and explains why certain elements are more reactive than others. Conversely, core electrons contribute to the overall atomic structure but do not directly influence an atom's reactivity or its tendency to form bonds. Understanding the distinction between valence and core electrons is essential for grasping various chemical principles, including electronegativity, ionization energy, and electron affinity. Electronegativity, for instance, is a measure of an atom's ability to attract valence electrons in a covalent bond, which is directly related to the number of valence electrons and their proximity to the nucleus. Similarly, ionization energy—the energy required to remove an electron from an atom—is significantly lower for valence electrons compared to core electrons due to their higher energy levels. In summary, while core electrons provide structural integrity and stability to an atom by filling inner shells, valence electrons are the key players in chemical bonding and reactivity. Their unique position in the outermost shell makes them crucial for understanding how atoms interact with each other to form molecules and compounds. This fundamental difference underscores why valence electrons are so important in chemistry and why their study is vital for comprehending a wide range of chemical phenomena.
The Significance of Valence Electrons in Chemical Bonding
Valence electrons, the outermost electrons of an atom, play a pivotal role in chemical bonding, determining how atoms interact and form compounds. These electrons are crucial for understanding the fundamental processes that govern the formation of ionic and covalent bonds. In ionic bonds, valence electrons are transferred between atoms, leading to the creation of ions with opposite charges that attract each other. Conversely, covalent bonds involve the sharing of valence electrons between atoms, resulting in a stable molecule. The number and arrangement of valence electrons also dictate an atom's bonding capacity, influencing its ability to form bonds with other atoms. This article delves into these key aspects, exploring the formation of ionic bonds, the formation of covalent bonds, and how valence electrons determine an atom's bonding capacity. By grasping these concepts, readers will gain a deeper understanding of the significance of valence electrons in chemical bonding, setting the stage for a comprehensive exploration of the subject. For a foundational understanding, it is essential to begin with **Understanding Valence Electrons: The Basics**.
Formation of Ionic Bonds
The formation of ionic bonds is a fundamental process in chemistry, underscored by the pivotal role of valence electrons. These bonds arise when there is a significant difference in the electronegativity between two atoms, typically involving a metal and a nonmetal. The process begins with the transfer of valence electrons from the metal atom, which has a relatively low ionization energy, to the nonmetal atom, which has a high electron affinity. This electron transfer results in the metal atom losing one or more electrons to form a positively charged ion (cation), while the nonmetal atom gains these electrons to form a negatively charged ion (anion). For instance, when sodium (Na) reacts with chlorine (Cl), sodium loses one valence electron to become Na⁺, and chlorine gains this electron to become Cl⁻. The electrostatic attraction between the oppositely charged ions then holds them together, forming an ionic bond. This transfer of valence electrons is crucial because it allows both atoms to achieve a stable electronic configuration, often mimicking the noble gas configuration. In the case of sodium and chlorine, sodium loses its single valence electron to achieve the stable configuration of neon, while chlorine gains an electron to achieve the stable configuration of argon. This stability is key to the formation and strength of the ionic bond. The significance of valence electrons in this process cannot be overstated; without their transfer, the ions would not form, and thus, no ionic bond would be established. Moreover, the nature of valence electrons influences the properties of the resulting ionic compound. For example, the lattice energy of an ionic compound, which is the energy required to separate the ions in the solid state, is directly related to the charge and size of the ions involved. Compounds with higher charges and smaller ion sizes tend to have higher lattice energies, indicating stronger ionic bonds. This, in turn, affects various physical properties such as melting point, boiling point, and solubility. In summary, the formation of ionic bonds is intricately linked with the behavior and transfer of valence electrons. These electrons are the key players in achieving stable electronic configurations for both metal and nonmetal atoms, leading to the creation of cations and anions that are electrostatically attracted to each other. Understanding this process highlights why valence electrons are so important in chemical bonding: they dictate not only the type of bond that forms but also the properties of the resulting compound. This fundamental concept underscores the significance of valence electrons in all aspects of chemical bonding and reactivity.
Formation of Covalent Bonds
The formation of covalent bonds is a fundamental process in chemistry, driven by the interactions between valence electrons. These bonds are crucial for the structure and stability of molecules, and their significance can be understood through the principles of valence electron behavior. Covalent bonds form when two or more atoms share one or more pairs of valence electrons to achieve a stable electronic configuration, often mimicking the noble gas configuration. This sharing occurs because atoms seek to fill their outermost energy level with a full complement of electrons, thereby reducing their potential energy and increasing stability. In a covalent bond, each atom contributes one or more valence electrons to the shared pair. For example, in the formation of a hydrogen molecule (H₂), each hydrogen atom has one valence electron. When these two atoms come together, they share their valence electrons to form a single covalent bond, resulting in a molecule where each hydrogen atom effectively has two electrons in its outer shell, similar to the noble gas helium. This sharing can be symmetrical, as in the case of H₂, or asymmetrical, leading to polar covalent bonds where the shared electrons are not equally distributed between the atoms. The type and number of covalent bonds an atom can form are determined by its valence electrons. For instance, carbon has four valence electrons and can form four covalent bonds, which is why it is so versatile in organic chemistry. Oxygen, with six valence electrons, typically forms two covalent bonds to achieve an octet configuration similar to neon. The ability of atoms to form multiple bonds (single, double, triple) also depends on their valence electron count and the need to achieve stability. The significance of valence electrons in covalent bonding extends beyond just the formation of bonds; it influences the shape and reactivity of molecules. The arrangement of valence electrons around an atom determines its electron geometry and molecular shape according to VSEPR theory (Valence Shell Electron Pair Repulsion). This theory explains how electron pairs arrange themselves to minimize repulsion, leading to specific shapes such as linear, trigonal planar, or tetrahedral. In summary, the formation of covalent bonds is a direct result of the interactions and sharing of valence electrons among atoms. Understanding these interactions is essential for comprehending the structure, stability, and reactivity of molecules. The role of valence electrons in determining the type and number of covalent bonds an atom can form underscores their importance in chemical bonding and highlights why valence electrons are a cornerstone of modern chemistry.
Determining Bonding Capacity
Determining bonding capacity is a crucial aspect of understanding chemical bonding, and it is fundamentally tied to the concept of valence electrons. Valence electrons, located in the outermost shell of an atom, are the electrons that participate in forming chemical bonds. The number and arrangement of these electrons dictate an atom's ability to form bonds with other atoms. To determine an atom's bonding capacity, one must first identify the number of valence electrons it possesses. For main group elements, this can be done by looking at the group number in the periodic table; for example, elements in Group 1 have one valence electron, while those in Group 17 have seven. The octet rule, which states that atoms tend to gain, lose, or share electrons to achieve a full outer shell with eight electrons (like the noble gases), is a key principle in predicting bonding behavior. For instance, hydrogen has one valence electron and typically forms one bond to achieve a stable configuration similar to helium. Carbon, with four valence electrons, can form four bonds to reach an octet. Oxygen, having six valence electrons, usually forms two bonds to complete its outer shell. By understanding these valence electron counts and their tendencies, chemists can predict the types and numbers of bonds an atom will form. Moreover, the shape and polarity of molecules are influenced by the bonding capacity determined by valence electrons. For example, in a water molecule (H₂O), oxygen's six valence electrons lead it to form two single bonds with hydrogen atoms and retain two lone pairs, resulting in a bent molecular geometry due to electron pair repulsion. In addition to predicting bond types and molecular shapes, knowing an atom's bonding capacity helps in understanding reactivity. Atoms with incomplete outer shells are more reactive because they seek to achieve stability through bonding. This reactivity is pivotal in various chemical reactions and processes, such as combustion reactions where oxygen's high bonding capacity makes it an essential reactant. In summary, determining an atom's bonding capacity through its valence electrons is essential for understanding chemical bonding. It allows chemists to predict the number and type of bonds an atom will form, the shape of molecules, and the reactivity of elements. This fundamental knowledge underpins much of modern chemistry and is crucial for advancing our understanding of chemical processes and reactions.
Valence Electrons and Their Impact on Chemical Properties
Valence electrons, the outermost electrons in an atom, play a pivotal role in determining the chemical properties of elements. These electrons are crucial for understanding how atoms interact with each other, forming bonds and participating in chemical reactions. The impact of valence electrons on reactivity is significant, as they dictate an atom's ability to gain, lose, or share electrons with other atoms. Additionally, valence electrons influence the electron affinity and ionization energy of an element, which are key factors in predicting its chemical behavior. Furthermore, the arrangement and number of valence electrons determine whether a material is conductive or insulating, affecting its electrical properties. This article delves into these aspects, exploring how valence electrons shape the reactivity, electron affinity, ionization energy, and conductivity of elements. By grasping these concepts, readers will gain a deeper understanding of the fundamental principles governing chemical interactions. To fully appreciate these complex relationships, it is essential to start with a solid foundation: Understanding Valence Electrons: The Basics.
Influence on Reactivity
The influence on reactivity is a critical aspect of understanding the role of valence electrons in chemical properties. Valence electrons, located in the outermost shell of an atom, are pivotal in determining how an atom interacts with other atoms to form chemical bonds. These electrons are highly reactive because they are involved in the formation of covalent, ionic, and metallic bonds. The number and arrangement of valence electrons dictate an atom's tendency to gain, lose, or share electrons, thereby influencing its reactivity. For instance, atoms with a full outer shell (like noble gases) are generally unreactive due to their stable electron configuration. Conversely, atoms with partially filled outer shells (such as alkali metals and halogens) are highly reactive. Alkali metals, with one valence electron, readily lose this electron to achieve a stable configuration, while halogens, needing one more electron to fill their outer shell, are eager to gain an electron. This propensity for gaining or losing electrons drives their reactivity and explains why these elements are often involved in chemical reactions. Moreover, the arrangement of valence electrons affects the types of bonds an atom can form. For example, carbon's four valence electrons allow it to form four covalent bonds, making it a versatile element capable of forming complex molecules like organic compounds. Similarly, oxygen's six valence electrons enable it to form two covalent bonds, which is crucial for its role in water (H₂O) and other biomolecules. The influence of valence electrons on reactivity also extends to the concept of electronegativity—the ability of an atom to attract electrons in a covalent bond. Atoms with high electronegativity values (like fluorine) pull electrons closer to themselves, affecting the polarity of bonds and influencing the overall reactivity of molecules. This polarity can lead to the formation of hydrogen bonds and other intermolecular forces that are essential for the properties of substances like water and biological molecules. In summary, the influence of valence electrons on reactivity is multifaceted and fundamental to understanding chemical behavior. By determining an atom's tendency to gain, lose, or share electrons and influencing bond types and electronegativity, valence electrons play a central role in shaping the chemical properties of elements and their compounds. This understanding is crucial for predicting and explaining various chemical phenomena, from simple reactions to complex biological processes.
Determination of Electron Affinity and Ionization Energy
Determination of Electron Affinity and Ionization Energy is a crucial aspect in understanding the chemical properties of elements, particularly when considering the role of valence electrons. Electron affinity, the energy change associated with the addition of an electron to a neutral atom, and ionization energy, the energy required to remove an electron from a neutral atom, are both directly influenced by the configuration and number of valence electrons. For instance, elements with a nearly full outer shell tend to have high electron affinities because adding an electron completes the shell, leading to a stable configuration. Conversely, elements with a nearly empty outer shell have low electron affinities as they are less inclined to accept additional electrons. Similarly, ionization energy is higher for elements with a full outer shell due to the strong attraction between the nucleus and the valence electrons, making it more difficult to remove an electron. In contrast, elements with fewer valence electrons have lower ionization energies as there is less nuclear attraction holding onto those electrons. The determination of these energies can be approached through various experimental and theoretical methods. Experimentally, techniques such as photoelectron spectroscopy can measure the energy required to remove an electron from an atom, providing direct data on ionization energy. Electron affinity can be determined indirectly by measuring the energy released when an electron is added to an atom, often through reactions involving the formation of anions. Theoretically, quantum mechanical models and computational chemistry can predict these values with high accuracy by simulating the electronic structure of atoms and molecules. Understanding electron affinity and ionization energy is vital because these properties significantly impact chemical reactivity. For example, metals typically have low ionization energies and low electron affinities, making them good electron donors in chemical reactions. Nonmetals, on the other hand, have high electron affinities and high ionization energies, making them good electron acceptors. This dichotomy underpins many fundamental chemical processes such as the formation of ionic bonds and the reactivity of elements in different groups of the periodic table. In summary, the determination of electron affinity and ionization energy provides critical insights into how valence electrons influence chemical behavior. These properties are essential for predicting how elements will react with each other and understanding the underlying reasons for their reactivity patterns. By grasping these concepts, chemists can better explain and predict a wide range of chemical phenomena, highlighting the importance of valence electrons in shaping the chemical properties of elements.
Role in Conductivity and Insulation
Valence electrons play a pivotal role in determining the conductivity and insulation properties of materials, making them a crucial aspect of understanding chemical properties. Conductivity, the ability of a material to allow the flow of electric current, is largely influenced by the availability and mobility of valence electrons. In metals, valence electrons are often delocalized, meaning they are not tightly bound to individual atoms but are free to move within the material. This delocalization allows these electrons to act as charge carriers, facilitating the flow of electricity. For instance, in copper, the valence electrons are highly mobile, making it an excellent conductor. Conversely, in insulators like glass or rubber, valence electrons are tightly bound to their respective atoms, preventing them from moving freely and thus inhibiting electrical conductivity. The arrangement and behavior of valence electrons also dictate the thermal conductivity of materials. In metals, the same delocalized electrons that contribute to electrical conductivity also facilitate the transfer of thermal energy. This is why metals generally have high thermal conductivity compared to insulators. On the other hand, insulators have valence electrons that are localized and do not participate in thermal energy transfer, resulting in lower thermal conductivity. In addition to metals and insulators, there is a third category known as semiconductors, where the role of valence electrons is more nuanced. Semiconductors have a narrow bandgap between their valence and conduction bands, allowing some valence electrons to be excited into the conduction band under certain conditions. This property makes semiconductors versatile for applications in electronics, as their conductivity can be controlled by introducing impurities (doping) that alter the number of available charge carriers. Moreover, the concept of valence electrons extends to understanding superconductors—materials that exhibit zero electrical resistance at very low temperatures. In these materials, the valence electrons form Cooper pairs, which can move through the material without resistance due to their collective behavior. This phenomenon highlights the complex and critical role that valence electrons play in determining the electrical properties of materials. In summary, the behavior and availability of valence electrons are fundamental in determining whether a material is a conductor, insulator, or semiconductor. Their mobility and interaction with other electrons and atoms dictate the material's ability to conduct electricity and heat, making them essential for understanding and predicting various chemical and physical properties. This underscores why valence electrons are so important in chemistry and materials science.