Why Does Electronegativity Increase Across A Period
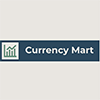
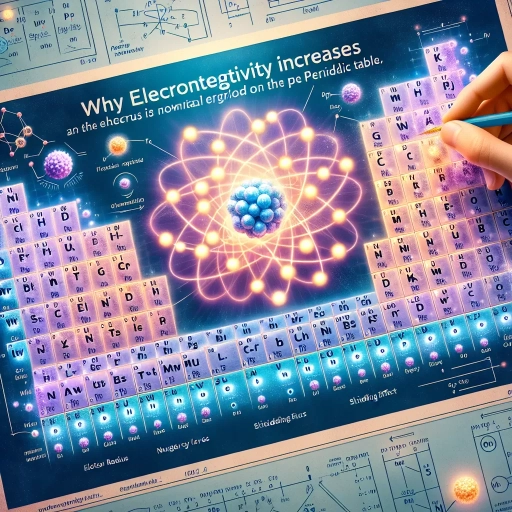
Electronegativity, a measure of an atom's ability to attract electrons in a covalent bond, exhibits a significant increase as you move across a period in the periodic table. This trend is not merely a coincidence but is rooted in several fundamental principles of atomic structure and chemistry. To understand why electronegativity increases across a period, it is crucial to delve into three key factors: atomic radius, effective nuclear charge, and electron configuration. Firstly, the decrease in atomic radius as you move across a period plays a pivotal role in enhancing electronegativity. As atoms become smaller, the distance between the nucleus and valence electrons decreases, allowing for a stronger attraction. Secondly, the effective nuclear charge—the net positive charge experienced by valence electrons—increases due to the addition of protons in the nucleus without a corresponding increase in shielding electrons. This heightened positive charge further pulls electrons closer, boosting electronegativity. Lastly, changes in electron configuration and the filling of valence shells also contribute to this trend by altering the distribution and availability of electrons for bonding. Understanding these factors is essential for grasping the underlying mechanisms driving the increase in electronegativity across a period. Let's begin by examining how atomic radius and its impact on electronegativity set the stage for this phenomenon.
Atomic Radius and Its Impact on Electronegativity
Atomic radius, a fundamental concept in chemistry, plays a crucial role in understanding the behavior of elements and their interactions. As we traverse the periodic table, particularly across a period, the atomic radius decreases due to the addition of electrons to the same principal energy level, leading to increased nuclear attraction. This decrease in atomic radius has significant implications for the electronegativity of elements. Electronegativity, the measure of an atom's ability to attract electrons in a covalent bond, is directly influenced by the proximity of electrons to the nucleus. When electrons are closer to the nucleus, the nuclear attraction enhances, resulting in higher electronegativity values. In this article, we will delve into the relationship between atomic radius and electronegativity, exploring how the decrease in atomic radius across a period, the increased proximity of electrons to the nucleus, and the enhanced nuclear attraction collectively impact an element's electronegativity. By examining these interconnected concepts, we aim to provide a comprehensive understanding of how atomic radius shapes the electronegative properties of elements.
Decrease in Atomic Radius Across a Period
As we traverse a period in the periodic table from left to right, a significant trend emerges: the atomic radius decreases. This phenomenon is crucial for understanding various chemical properties, including electronegativity. The decrease in atomic radius across a period can be attributed to the increasing effective nuclear charge and the addition of electrons to the same principal energy level. When moving from one element to the next within a period, an additional proton is added to the nucleus, which enhances the nuclear charge. This increased positive charge pulls the electrons closer to the nucleus, resulting in a tighter hold on them. Simultaneously, electrons are added to the same principal energy level (or shell), but these electrons do not shield each other effectively due to their similar energies. Consequently, the effective nuclear charge felt by each electron increases, leading to a stronger attraction between the nucleus and the electrons. This stronger attraction causes the electron cloud to contract, reducing the distance between the nucleus and the outermost electrons. As a result, the atomic radius decreases as we move across a period. For instance, in the second period, lithium (Li) has an atomic radius of about 152 pm, while neon (Ne) at the end of the period has an atomic radius of approximately 51 pm. The decrease in atomic radius has profound implications for chemical behavior. Smaller atoms are more electronegative because their electrons are held more tightly by the nucleus. This tighter hold makes it more difficult for other atoms to attract these electrons, thereby increasing the atom's electronegativity. Consequently, as we move across a period, elements become more electronegative due to their smaller atomic radii and increased effective nuclear charge. Understanding this trend is essential for predicting chemical reactivity and bond formation. For example, fluorine (F), with its small atomic radius and high electronegativity, forms strong covalent bonds and is highly reactive compared to sodium (Na), which has a larger atomic radius and lower electronegativity. The interplay between atomic radius and electronegativity underscores the fundamental principles governing chemical interactions and reactivity patterns across the periodic table. In summary, the decrease in atomic radius across a period is a direct consequence of increasing effective nuclear charge and the addition of electrons to the same energy level. This reduction in size enhances electronegativity, influencing how elements interact chemically and form bonds. Recognizing this relationship is vital for comprehending the intricate dynamics of atomic structure and its impact on chemical properties.
Increased Proximity of Electrons to the Nucleus
As we delve into the intricacies of atomic structure and its influence on chemical properties, the concept of increased proximity of electrons to the nucleus emerges as a pivotal factor. This phenomenon is closely tied to the atomic radius and its impact on electronegativity, particularly when examining trends across a period in the periodic table. When moving from left to right across a period, the atomic number increases, which means that each successive element has one more proton in its nucleus. This increase in nuclear charge exerts a stronger electrostatic pull on the electrons, drawing them closer to the nucleus. Consequently, the atomic radius decreases because the electrons are more tightly bound, resulting in a smaller overall size of the atom. This reduced atomic radius has a direct and significant impact on electronegativity. Electronegativity is a measure of an atom's ability to attract electrons in a covalent bond. As electrons are drawn closer to the nucleus due to the increased nuclear charge, the atom's ability to attract additional electrons also increases. This heightened attraction is what drives the increase in electronegativity across a period. For instance, consider the elements in the second period of the periodic table: lithium (Li), beryllium (Be), boron (B), carbon (C), nitrogen (N), oxygen (O), fluorine (F), and neon (Ne). As you move from lithium to neon, each element's atomic radius decreases due to the increasing nuclear charge, leading to a corresponding increase in electronegativity. Fluorine, with its high electronegativity value, exemplifies this trend; it has a strong tendency to attract electrons because its electrons are tightly bound close to its nucleus. In summary, the increased proximity of electrons to the nucleus is a fundamental reason why electronegativity increases across a period. This trend is underpinned by the decrease in atomic radius as nuclear charge increases, enhancing an atom's ability to attract electrons and thus elevating its electronegativity. Understanding this relationship is crucial for predicting chemical behavior and forming bonds between atoms, making it a cornerstone of modern chemistry.
Enhanced Nuclear Attraction
Enhanced nuclear attraction plays a pivotal role in understanding the trend of increasing electronegativity across a period in the periodic table. As atomic radius decreases from left to right across a period, the distance between the nucleus and the valence electrons diminishes. This reduction in atomic radius results in a stronger electrostatic pull exerted by the nucleus on the electrons, a phenomenon known as enhanced nuclear attraction. Consequently, the nucleus holds onto the electrons more tightly, making it more difficult for other atoms to attract these electrons. This heightened nuclear-electron interaction is crucial because it directly influences the electronegativity of an element. Electronegativity, a measure of an atom's ability to attract electrons in a covalent bond, increases as the atomic radius decreases due to this enhanced nuclear attraction. The smaller atomic radius means that the valence electrons are closer to the nucleus, which has a greater positive charge relative to the number of electrons. This increased proximity amplifies the electrostatic force between the nucleus and the valence electrons, thereby enhancing the atom's ability to attract additional electrons. For instance, as you move from sodium (Na) to neon (Ne) across period 3, the atomic radius decreases significantly, leading to a substantial increase in electronegativity. Neon, with its smaller atomic radius and thus stronger nuclear-electron attraction, exhibits higher electronegativity compared to sodium. Moreover, enhanced nuclear attraction is not just a simple geometric effect but also involves changes in the effective nuclear charge experienced by the valence electrons. As you move across a period, each successive element adds one proton to the nucleus without adding a new energy level (or shell), which means that the effective nuclear charge increases. This increase in effective nuclear charge further strengthens the attraction between the nucleus and the valence electrons, contributing to higher electronegativity values. In summary, enhanced nuclear attraction is a fundamental concept that underpins the increase in electronegativity across a period. The decrease in atomic radius and the corresponding increase in effective nuclear charge result in a stronger electrostatic pull on the valence electrons, making it easier for atoms to attract additional electrons and thus increasing their electronegativity. This understanding is essential for predicting chemical behavior and explaining various trends observed in the periodic table.