When Will Winter End In Canada
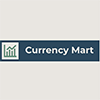
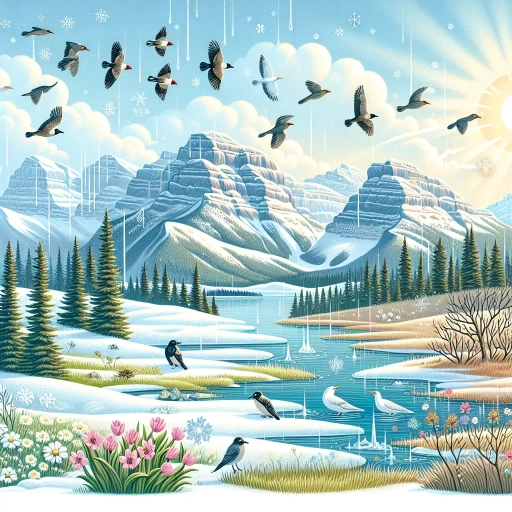
As the chill of winter grips Canada, many residents eagerly await the arrival of warmer days. But when exactly can Canadians expect the end of winter? The answer is not as straightforward as it might seem, given the country's vast and diverse climate zones. To understand when winter will end, it is crucial to delve into several key areas. First, we must **Understand Canada's Climate Zones**, as the timing of winter's end varies significantly from coast to coast. Next, examining **Historical Trends and Patterns** can provide valuable insights into how past winters have behaved and what this might mean for the current season. Finally, **Predicting Winter's End with Modern Tools** involves leveraging advanced meteorological techniques and technologies to forecast the exact date of winter's conclusion. By exploring these aspects, we can gain a comprehensive understanding of when Canadians can bid farewell to the cold and welcome the onset of spring. Let's begin by **Understanding Canada's Climate Zones**.
Understanding Canada's Climate Zones
Canada, known for its vast and diverse geography, encompasses a wide range of climate zones that significantly influence the country's ecosystems, economies, and daily life. Understanding these climate zones is crucial for appreciating the complexities of Canada's environment. This article delves into the intricacies of Canada's climate, focusing on three key aspects: Regional Variations in Winter Duration, the Impact of Latitude on Winter Length, and the Role of Coastal vs. Inland Areas. By examining these factors, we can gain a deeper insight into how different regions of Canada experience distinct climatic conditions. For instance, the duration of winter varies dramatically across different regions, with some areas experiencing long, harsh winters while others enjoy milder conditions. Latitude plays a pivotal role in determining the length of winter, with northern regions typically experiencing longer and colder winters compared to their southern counterparts. Additionally, the distinction between coastal and inland areas highlights how proximity to large bodies of water can moderate temperatures and precipitation patterns. Understanding these variations is essential for comprehending the broader context of Canada's climate zones, which is the central theme of this article. By exploring these elements, we aim to provide a comprehensive overview of Understanding Canada's Climate Zones.
Regional Variations in Winter Duration
Regional variations in winter duration are a significant aspect of Canada's diverse climate landscape, reflecting the country's vast geographical expanse and varied topography. From the mild winters of British Columbia to the harsh, prolonged winters of the Arctic territories, each region experiences winter in a unique way. In Western Canada, particularly in British Columbia and parts of Alberta, winters are generally shorter and milder due to the moderating influence of the Pacific Ocean. Here, temperatures rarely drop below freezing for extended periods, and snowfall is often minimal compared to other parts of the country. In contrast, Central Canada, including provinces like Ontario and Quebec, experiences a more traditional winter with cold temperatures and significant snowfall. Winters in these regions can last from late November to early April, with January typically being the coldest month. The Great Lakes play a crucial role in moderating temperatures but also contribute to lake-effect snowfall, which can significantly impact local weather patterns. Eastern Canada, particularly the Maritime provinces, has a somewhat milder winter compared to Central Canada but still faces cold temperatures and considerable snowfall. The Atlantic Ocean's influence helps keep temperatures relatively stable, though storms from the Atlantic can bring heavy snow and ice. The Prairie provinces—Manitoba, Saskatchewan, and Alberta—experience some of the longest and coldest winters in Canada. These regions are far from any moderating oceanic influence, leading to extreme temperature fluctuations and prolonged periods of cold weather. Winters here can start as early as October and last until March or April. In Northern Canada, including Yukon, Northwest Territories, and Nunavut, winters are the longest and most severe. Here, the Arctic climate prevails with extended periods of sub-zero temperatures and limited daylight during the winter months. In some areas, winter can last from September to May or even longer. Understanding these regional variations is essential for predicting when winter will end in different parts of Canada. While general trends can be observed, local conditions such as proximity to large bodies of water or elevation can significantly impact the duration and intensity of winter. This knowledge helps Canadians prepare for seasonal changes and plan accordingly for activities ranging from agriculture to recreation. By recognizing these regional differences, individuals can better navigate Canada's diverse climate zones and make informed decisions about their daily lives during the winter months.
Impact of Latitude on Winter Length
The impact of latitude on winter length is a critical factor in understanding Canada's diverse climate zones. As one moves northward, the duration and severity of winter significantly increase due to the Earth's tilt and the resulting distribution of solar radiation. In Canada, this phenomenon is particularly pronounced. At lower latitudes, such as in Southern Ontario and British Columbia, winters are generally milder and shorter, with temperatures often remaining above freezing for longer periods. In contrast, regions at higher latitudes like Yukon, Nunavut, and parts of Northern Quebec experience extended and harsher winters. Here, the sun's rays strike the Earth at a more oblique angle, leading to reduced solar energy input and prolonged periods of cold temperatures. This latitudinal variation affects not only the length but also the intensity of winter. For instance, communities in the Arctic Circle may endure up to six months of winter, characterized by extreme cold and limited daylight. Conversely, areas closer to the 49th parallel enjoy relatively shorter winters with more moderate temperatures. The difference in winter duration also influences local ecosystems and human activities. In northern regions, the extended winter necessitates specialized adaptations such as insulated housing, snowmobile transportation, and unique agricultural practices. In contrast, southern regions can support a wider range of agricultural activities and outdoor recreational pursuits during the winter months. Understanding these latitudinal effects is essential for predicting when winter will end in different parts of Canada. While southern provinces may see the first signs of spring as early as late February or early March, northern territories often remain in winter's grip until late April or even May. This knowledge is crucial for planning agricultural cycles, managing natural resources, and ensuring public safety during severe weather conditions. Furthermore, it highlights the importance of regional climate considerations in policy-making and daily life across Canada's vast and climatically diverse landscape. In summary, the impact of latitude on winter length is a defining characteristic of Canada's climate zones. From the mild winters of the south to the prolonged and harsh conditions of the north, this factor shapes both environmental conditions and human experiences. Recognizing these differences is vital for understanding and adapting to Canada's varied climate, ensuring that residents and visitors alike are prepared for the unique challenges and opportunities presented by each region's distinct winter season.
Role of Coastal vs. Inland Areas
In the vast and diverse landscape of Canada, the distinction between coastal and inland areas plays a pivotal role in shaping the country's climate zones. Coastal regions, such as those along the Atlantic, Pacific, and Arctic coasts, are significantly influenced by the moderating effects of large bodies of water. These areas tend to experience milder temperatures compared to their inland counterparts due to the thermal mass of the oceans, which absorbs and releases heat slowly. This results in fewer extreme temperature fluctuations, leading to cooler summers and warmer winters. For instance, cities like Vancouver and Halifax enjoy relatively mild winters and cool summers, making them more hospitable year-round. Additionally, coastal areas often receive higher levels of precipitation, contributing to lush vegetation and rich biodiversity. In contrast, inland regions are characterized by more pronounced seasonal variations. Without the moderating influence of the ocean, these areas experience colder winters and hotter summers. Cities like Winnipeg and Calgary can see temperatures drop below -20°C in winter and rise above 30°C in summer. The lack of maritime influence also means that inland areas generally receive less precipitation, leading to drier conditions and different types of vegetation. For example, the Prairies are known for their vast expanses of grasslands and agricultural lands, which thrive under the continental climate conditions. Understanding these differences is crucial for predicting weather patterns and seasonal changes across Canada. For instance, knowing that coastal areas will likely remain warmer during winter helps residents prepare for less severe cold snaps. Conversely, inland residents must be prepared for harsher winter conditions and potential extreme weather events such as blizzards or heatwaves. This knowledge also informs agricultural practices, urban planning, and even tourism strategies, as different regions offer unique seasonal experiences. Moreover, the interplay between coastal and inland climates contributes to Canada's rich ecological diversity. The transition zones between these two types of climates support a wide range of ecosystems, from temperate rainforests near the coast to boreal forests further inland. This diversity not only enhances biodiversity but also provides various natural resources that are integral to Canada's economy. In summary, the distinction between coastal and inland areas is fundamental to understanding Canada's climate zones. While coastal regions enjoy milder temperatures and higher precipitation due to oceanic influences, inland areas experience more extreme seasonal variations. Recognizing these differences is essential for adapting to local climate conditions, managing natural resources effectively, and appreciating the unique characteristics of each region within Canada's vast and varied landscape.
Historical Trends and Patterns
Understanding historical trends and patterns in climate is crucial for grasping the complexities of our environment. This article delves into three key areas that provide a comprehensive insight into these trends. First, we explore **Long-term Climate Data Analysis**, examining decades of data to identify consistent changes and fluctuations in temperature, precipitation, and other climatic factors. Next, we discuss **Seasonal Shifts Over the Years**, highlighting how the timing and intensity of seasons have evolved, impacting ecosystems and human activities. Additionally, we analyze **Notable Weather Events and Their Impact**, focusing on significant storms, droughts, and other extreme weather phenomena that have shaped our understanding of climate variability. By examining these historical trends and patterns, we gain a deeper understanding of the dynamics that influence our planet's climate, ultimately leading to a more informed perspective on **Understanding Canada's Climate Zones**. This knowledge is essential for predicting future climate changes and making informed decisions about environmental management and policy.
Long-term Climate Data Analysis
Long-term climate data analysis is a crucial component in understanding historical trends and patterns, particularly when predicting the end of winter in Canada. By examining decades of climate data, researchers can identify significant shifts and cycles that influence seasonal transitions. This involves scrutinizing temperature records, precipitation patterns, and other meteorological indicators over extended periods. For instance, analyzing temperature trends from the past century reveals that Canada has experienced a gradual warming trend, especially in the winter months. This warming is more pronounced in the Arctic regions, where temperatures have risen at a rate twice as fast as the global average. Historical climate data also highlight the impact of natural climate variability such as the North Atlantic Oscillation (NAO) and the El Niño-Southern Oscillation (ENSO), which can significantly affect winter duration and severity. The NAO, for example, influences the strength of the westerly winds across the North Atlantic, impacting the trajectory of winter storms and cold air masses into Canada. Similarly, ENSO events can alter global atmospheric circulation patterns, leading to warmer or cooler winters depending on the phase of the cycle. Moreover, long-term data analysis helps in identifying regional differences within Canada. The country's vast geography means that winter patterns vary significantly from coast to coast. For example, British Columbia tends to have milder winters compared to provinces like Quebec and Ontario, which experience colder and longer winters. By analyzing these regional differences over time, scientists can better understand how local climate conditions are evolving. Advanced statistical techniques and machine learning algorithms are increasingly being used to analyze these extensive datasets. These methods allow for the detection of subtle patterns and correlations that might not be apparent through traditional analysis. For instance, machine learning models can predict the likelihood of an early or late winter based on historical data and current climate conditions. In addition to these technical approaches, long-term climate data analysis also informs policy and decision-making. Understanding historical trends helps in developing more accurate forecasts, which are essential for agriculture, transportation, and public health sectors. For example, knowing when winter is likely to end can help farmers plan planting schedules and municipalities prepare for spring maintenance. In conclusion, long-term climate data analysis is indispensable for comprehending historical trends and patterns that shape Canada's winter seasons. By leveraging this data, scientists can provide insights into when winter might end, helping various stakeholders prepare for the transition into spring. This integrated approach ensures that our understanding of climate dynamics is both robust and actionable, enabling better adaptation to changing environmental conditions.
Seasonal Shifts Over the Years
Seasonal shifts over the years have been a subject of keen interest and study, particularly in regions like Canada where the climate is known for its pronounced seasonal variations. Historically, these shifts have been influenced by a combination of natural and anthropogenic factors. Natural climate variability, such as the El Niño-Southern Oscillation (ENSO) and the North Atlantic Oscillation (NAO), has long played a role in altering seasonal patterns. For instance, during El Niño events, Canada often experiences warmer winters due to increased atmospheric moisture and altered jet stream patterns. However, in recent decades, the impact of climate change has become increasingly evident. Rising global temperatures, primarily driven by greenhouse gas emissions, have led to more frequent and severe weather events. This is particularly noticeable in Canada where winters are becoming shorter and milder. Studies indicate that the onset of spring is occurring earlier, while the onset of autumn is delayed, resulting in longer growing seasons but also more unpredictable weather conditions. One of the most significant historical trends observed is the reduction in snow cover and ice extent. In regions like Quebec and Ontario, snow cover has decreased by up to 20% over the past few decades. This reduction not only affects local ecosystems but also has economic implications for industries such as winter tourism and agriculture. Additionally, changes in precipitation patterns are altering the distribution and intensity of seasonal rainfall and snowfall, leading to more frequent flooding in spring and droughts in summer. The historical data also show that these seasonal shifts are not uniform across Canada. The Arctic regions are experiencing some of the most rapid changes, with temperatures rising at rates twice as fast as the global average. This has profound implications for indigenous communities, wildlife habitats, and global climate systems. In contrast, southern regions like British Columbia are seeing less dramatic changes but still face challenges such as increased risk of wildfires during longer dry seasons. Understanding these historical trends and patterns is crucial for predicting future seasonal shifts and preparing for their impacts. By analyzing long-term data sets and incorporating climate models, scientists can provide insights into when winter might end in different parts of Canada. This information is vital for planning agricultural cycles, managing water resources, and ensuring public safety during extreme weather events. As Canada continues to navigate the complexities of a changing climate, recognizing and adapting to these seasonal shifts will be essential for sustainable development and environmental stewardship.
Notable Weather Events and Their Impact
Notable weather events have significantly shaped historical trends and patterns, particularly in Canada where the climate is known for its variability and extremes. One of the most impactful events in recent history is the 1998 Ice Storm, which devastated parts of Quebec and Ontario. This storm, lasting several days, brought down power lines and left millions without electricity, highlighting the vulnerability of infrastructure to severe weather conditions. The economic impact was substantial, with damages estimated in the billions of dollars, and it led to significant improvements in emergency preparedness and grid resilience. Another notable event is the 2013 Alberta Floods, which saw unprecedented rainfall cause widespread flooding in Calgary and surrounding areas. This disaster underscored the importance of floodplain management and prompted major investments in flood mitigation infrastructure. The floods also highlighted the role of climate change in exacerbating extreme weather events, as warmer temperatures can lead to more intense precipitation. The 2019 Polar Vortex event brought record-low temperatures to much of Canada, particularly in the provinces of Manitoba and Ontario. This extreme cold snap resulted in numerous fatalities and widespread disruptions to daily life, emphasizing the need for robust public health measures during such events. It also highlighted the interconnectedness of global weather patterns, as the polar vortex was influenced by changes in the Arctic climate. Hurricane Igor in 2010 had a profound impact on Newfoundland and Labrador, causing severe damage and flooding along the eastern coast. This event underscored the importance of coastal resilience and emergency preparedness in regions prone to hurricane landfalls. It also led to enhanced meteorological forecasting capabilities to better predict and prepare for such storms. These notable weather events not only reflect historical trends but also inform future climate projections. They demonstrate how extreme weather can have far-reaching consequences on infrastructure, public health, and economic stability. By studying these events, scientists and policymakers can better understand climate patterns and develop strategies to mitigate the impacts of future weather extremes, ultimately contributing to a more resilient and prepared society as Canada navigates the challenges of climate change. These insights are crucial for predicting when winter will end in Canada, as understanding historical weather patterns helps in forecasting future trends and preparing for potential extremes.
Predicting Winter's End with Modern Tools
As the chill of winter begins to wane, predicting its end has become increasingly crucial for various sectors, from agriculture to urban planning. Modern tools have revolutionized our ability to forecast the transition from winter to spring, offering unprecedented accuracy and detail. This article delves into the cutting-edge methods that enable us to predict winter's end with greater precision. We explore the use of meteorological models and forecasts, which leverage complex algorithms and real-time data to provide reliable predictions. Additionally, we examine the role of satellite imaging and remote sensing, which offer a bird's-eye view of climatic changes and ground conditions. Furthermore, we discuss the impact of climate change on these predictions, highlighting how shifting global weather patterns affect our ability to forecast seasonal transitions. By understanding these advanced tools and their applications, we can better navigate the complexities of predicting winter's end, ultimately enhancing our comprehension of Canada's diverse climate zones.
Use of Meteorological Models and Forecasts
Predicting the end of winter in Canada relies heavily on the sophisticated use of meteorological models and forecasts. These tools are crucial for understanding and anticipating weather patterns, enabling accurate predictions that help in planning and decision-making. Meteorological models, such as the Global Forecast System (GFS) and the European Centre for Medium-Range Weather Forecasts (ECMWF) model, utilize complex algorithms and vast datasets to simulate atmospheric conditions. These models integrate various parameters including temperature, humidity, wind patterns, and atmospheric pressure to generate detailed forecasts. Advanced forecasting techniques involve ensemble modeling, where multiple runs of a model are conducted with slight variations in initial conditions to account for uncertainties. This approach provides a range of possible outcomes, allowing meteorologists to assess the likelihood of different scenarios. Additionally, high-resolution models like the Weather Research and Forecasting (WRF) model offer detailed insights into local weather phenomena, which are particularly useful for predicting regional variations in weather patterns. Satellite imagery and radar data also play a pivotal role in enhancing forecast accuracy. Satellites in orbit around the Earth capture high-resolution images of cloud formations, precipitation patterns, and other atmospheric conditions. This real-time data is fed into the models to improve their predictive capabilities. Radar systems on the ground provide detailed information about precipitation intensity and movement, further refining the forecasts. The integration of these tools with machine learning algorithms has revolutionized the field of meteorology. Machine learning models can analyze historical data and identify patterns that may not be immediately apparent to human analysts. This synergy between traditional modeling techniques and modern computational methods significantly enhances the accuracy and reliability of weather forecasts. In the context of predicting winter's end in Canada, these advanced meteorological models and forecasts are indispensable. They help in identifying the transition from winter to spring by tracking changes in temperature trends, the melting of snow cover, and shifts in atmospheric circulation patterns. By leveraging these sophisticated tools, meteorologists can provide timely and accurate information to the public, aiding in preparations for the upcoming season and supporting critical decision-making in sectors such as agriculture, transportation, and emergency management. Ultimately, the precise use of meteorological models and forecasts ensures that Canadians are well-prepared for the end of winter and the onset of spring.
Role of Satellite Imaging and Remote Sensing
The role of satellite imaging and remote sensing is pivotal in predicting the end of winter, particularly in regions like Canada where harsh and variable weather conditions prevail. These advanced technologies enable scientists to monitor and analyze vast areas of land and atmospheric conditions with unprecedented precision. Satellite imaging captures high-resolution images of the Earth's surface, allowing for the detection of subtle changes in snow cover, ice extent, and vegetation health. This data is crucial for tracking the onset of spring, as it provides real-time insights into the melting of snow and ice, which are key indicators of winter's end. Remote sensing, on the other hand, involves the use of sensors to gather data about the Earth's surface without physical contact. These sensors can measure various parameters such as temperature, moisture levels, and spectral reflectance, which are essential for understanding environmental changes. By analyzing these data sets, researchers can identify patterns and trends that signal the transition from winter to spring. For instance, increased vegetation reflectance in the visible spectrum can indicate the start of plant growth, while changes in soil moisture levels can suggest thawing permafrost. Moreover, satellite imaging and remote sensing technologies are integrated with other modern tools such as GIS (Geographic Information Systems) and machine learning algorithms to enhance predictive capabilities. These integrations allow for the creation of detailed maps and models that forecast when winter will end based on historical data and current environmental conditions. This integrated approach not only improves the accuracy of predictions but also provides valuable information for various stakeholders, including farmers, urban planners, and emergency responders who need to prepare for seasonal changes. In Canada, where winters can be particularly long and severe, the application of satellite imaging and remote sensing is especially beneficial. These technologies help in monitoring large areas that are difficult to access on the ground, such as remote wilderness regions or vast agricultural lands. By leveraging these tools, scientists can provide timely and accurate forecasts that help communities prepare for the end of winter, facilitating smoother transitions into spring and ensuring better resource management and planning. In summary, satellite imaging and remote sensing play a critical role in predicting when winter will end by providing comprehensive and real-time data on environmental changes. These technologies, when combined with other modern tools, offer a robust framework for accurate forecasting, benefiting both scientific research and practical applications across diverse sectors in Canada.
Impact of Climate Change on Predictions
The impact of climate change on weather predictions, particularly in forecasting the end of winter in Canada, is multifaceted and profound. Rising global temperatures due to climate change alter the dynamics of atmospheric circulation, leading to more unpredictable and extreme weather patterns. This variability complicates the accuracy of traditional forecasting models, which often rely on historical data that may no longer be representative of current conditions. For instance, warmer winters can result in earlier thaws, but they also increase the likelihood of late-season cold snaps, making it challenging to pinpoint exactly when winter will end. Additionally, climate change influences the jet stream, a key factor in determining weather patterns over North America. A more meridional (wavy) jet stream can lead to prolonged periods of either cold or warm weather, further complicating predictions. Advanced modern tools, such as high-resolution climate models and machine learning algorithms, are being developed to better account for these changes. These tools integrate real-time data from a variety of sources, including satellite imagery and ground-based sensors, to provide more accurate and dynamic forecasts. However, even with these advancements, the inherent unpredictability introduced by climate change means that predicting the exact end of winter remains a complex task. As a result, meteorologists must continually adapt their methods and models to keep pace with the evolving climate, ensuring that Canadians receive the most reliable information possible about when winter will finally come to an end. This adaptability is crucial not only for everyday planning but also for long-term decision-making in agriculture, transportation, and other sectors heavily influenced by seasonal changes. In essence, while climate change presents significant challenges to predicting winter's end, the integration of modern tools and ongoing research in meteorology offer promising avenues for improving forecast accuracy and helping Canadians prepare for the uncertainties of a changing climate.