When The Moon Hatched
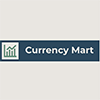
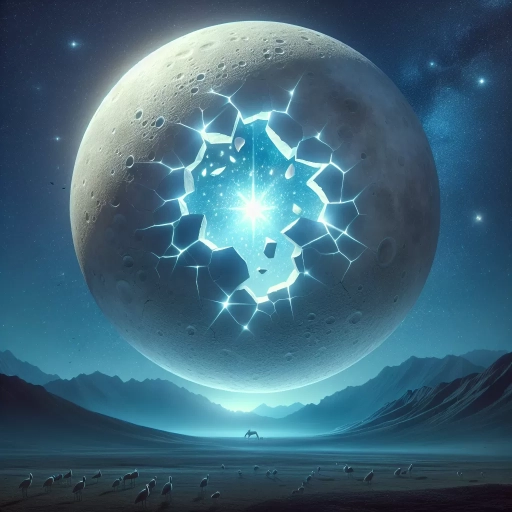
The moon, our closest celestial neighbor, has long fascinated humanity with its mysterious allure and significant influence on Earth's tides and night sky. But have you ever wondered when and how this lunar companion came into existence? The origin of the moon is a complex and intriguing topic that has captivated scientists and theorists for centuries. To delve into this enigma, we must first understand the moon's formation, a process that is deeply rooted in the early history of our solar system. This article will explore the scientific evidence and theories that shed light on the moon's birth, as well as the timeline and key events that have shaped its evolution over billions of years. By examining these aspects, we can gain a deeper understanding of how our moon hatched from the primordial chaos of space. Let us begin by understanding the moon's formation, a crucial step in unraveling the mysteries of our lunar companion.
Understanding the Moon's Formation
The Moon, Earth's closest celestial companion, has long fascinated humans with its enigmatic presence and pivotal role in our planet's history. Understanding the Moon's formation is a complex and intriguing topic that has garnered significant scientific attention. At the heart of this inquiry lies the Giant Impact Hypothesis, which posits that the Moon was formed from debris left over after a massive collision between Earth and a Mars-sized object known as Theia. This hypothesis is complemented by the processes of accretion and differentiation, which explain how the Moon's material coalesced and evolved into its current structure. Additionally, examining the early lunar environment provides insights into the Moon's surface and internal development, shedding light on its geological and atmospheric evolution. By delving into these three key areas—The Giant Impact Hypothesis, accretion and differentiation, and the early lunar environment—we can gain a comprehensive understanding of the Moon's formation, unraveling the mysteries that have captivated scientists and the public alike for centuries. Understanding the Moon's formation not only enriches our knowledge of our solar system but also deepens our appreciation for the intricate history of our celestial neighborhood.
The Giant Impact Hypothesis
The Giant Impact Hypothesis is a cornerstone theory in understanding the Moon's formation, offering a compelling narrative that has garnered significant scientific support. This hypothesis posits that the Moon was created as a result of a massive collision between Earth and a Mars-sized object known as Theia, which is believed to have existed in the early days of the solar system. This cataclysmic event, occurring approximately 4.5 billion years ago, is thought to have been so violent that it caused a substantial portion of Earth's mantle and crust to be ejected into space. Over time, these ejected materials coalesced under the influence of gravity, eventually forming the Moon. Key evidence supporting this hypothesis includes similarities in the chemical compositions of Earth and Moon rocks, particularly in terms of isotopic signatures. For instance, the Moon's rocks have identical oxygen isotope ratios to those found on Earth, suggesting a common origin. Additionally, the Moon's iron-depleted composition aligns with the idea that it formed primarily from Earth's mantle rather than its iron-rich core. The Moon's relatively small size compared to Earth also fits this model, as it would have formed from material ejected during such an impact rather than being captured from elsewhere in the solar system. Furthermore, computer simulations have successfully replicated the dynamics of such an impact, demonstrating how debris could have been ejected into orbit and subsequently coalesced into the Moon. These simulations also help explain why the Moon has a similar but distinct composition compared to Earth, reflecting the specific conditions under which it formed. The Giant Impact Hypothesis not only provides a coherent explanation for the Moon's origin but also sheds light on the early violent history of our solar system, where planetary collisions were more frequent and played a crucial role in shaping planetary bodies. In summary, the Giant Impact Hypothesis offers a robust and well-supported explanation for the Moon's formation. By integrating geological, isotopic, and computational evidence, this theory provides a comprehensive understanding of how our closest celestial neighbor came into existence. It underscores the dynamic and often violent processes that have sculpted our solar system over billions of years, making it an essential component of any discussion on lunar origins.
Accretion and Differentiation
**Accretion and Differentiation: The Moon's Formative Processes** The formation of the Moon is intricately linked to two fundamental processes: accretion and differentiation. Accretion, the gradual accumulation of material through collisions, played a pivotal role in the Moon's early development. Following the giant impact hypothesis, it is believed that a massive object, sometimes referred to as Theia, collided with Earth approximately 4.5 billion years ago. This catastrophic event ejected a significant portion of Earth's mantle and crust into space, where it coalesced into the Moon. As these debris particles collided and merged, they formed larger bodies known as planetesimals, which eventually grew into the Moon through continued accretion. Differentiation, the process by which a planet or moon separates into distinct layers based on density, was crucial in shaping the Moon's internal structure. As the Moon grew in size, its interior heated up due to the energy released from accretionary impacts and radioactive decay. This heat caused the Moon's material to melt partially, leading to the separation of heavier elements like iron and nickel from lighter silicate minerals. The denser metallic components sank to the center, forming a small iron-rich core, while the lighter silicates rose to form the crust and mantle. This differentiation process is evident in the Moon's composition, with its crust primarily composed of feldspar-rich rocks and its mantle made up of olivine and pyroxene minerals. Understanding these processes is essential for grasping how the Moon evolved from a chaotic, fragmented state into a cohesive, structured body. The accretion phase set the stage for differentiation by providing the necessary energy and material for the Moon's internal rearrangement. The resulting layered structure has been confirmed by seismic data from moonquakes, which reveal a crust, mantle, and small core. This internal differentiation also influenced the Moon's surface geology, with the crust being subjected to extensive magmatic and tectonic activities that shaped its characteristic maria and highlands. In summary, accretion and differentiation were the driving forces behind the Moon's formation and evolution. These processes not only determined the Moon's size and composition but also its internal and external structures, ultimately transforming it into the celestial body we observe today. By studying these formative stages, scientists can better understand how other moons and planets in our solar system may have formed, providing valuable insights into the broader context of planetary formation and evolution.
Early Lunar Environment
The early lunar environment was a complex and dynamic system that played a crucial role in the Moon's formation and evolution. Following the giant impact hypothesis, which suggests that the Moon was formed from debris left over after a massive collision between Earth and a Mars-sized object known as Theia, the lunar environment underwent significant transformations. Initially, the Moon is believed to have been in a molten state, with temperatures soaring due to the immense energy released during the impact. This period, known as the "magma ocean," saw the differentiation of the Moon's crust, mantle, and core as heavier elements like iron sank to the center while lighter materials rose to the surface. As the Moon cooled, it transitioned from a completely molten state to one with a solid crust. This process was marked by extensive volcanic activity, resulting in the formation of large dark basaltic plains known as "maria," which are visible from Earth. These maria are rich in iron and magnesium and were created when magma rose from the Moon's interior and solidified on its surface. The early lunar environment also experienced intense bombardment by asteroids and comets, leading to the formation of numerous craters that dot the Moon's surface today. The early atmosphere of the Moon was likely very different from what it is now; it may have included gases such as helium, neon, and argon released during volcanic activity. However, this atmosphere was short-lived due to the Moon's weak gravitational pull, which prevented it from retaining these gases. The absence of a significant atmosphere meant that there was no protection against solar wind or meteorite impacts, contributing to the Moon's barren and airless surface. Understanding these early conditions is crucial for piecing together the Moon's history and its relationship with Earth. For instance, studies of lunar rocks brought back by Apollo missions have provided valuable insights into the Moon's composition and thermal history. These findings support the giant impact hypothesis and highlight how the early lunar environment shaped the Moon into what we see today—a rocky, airless satellite with a rich geological past. In summary, the early lunar environment was characterized by extreme heat, extensive volcanic activity, and intense bombardment. These factors contributed to the Moon's unique geological features and its current state as a barren yet fascinating celestial body. By examining this period in detail, scientists can better understand not only how the Moon formed but also how it has evolved over billions of years to become an integral part of our Earth-Moon system.
Scientific Evidence and Theories
The formation of the Moon has long been a subject of intense scientific inquiry, with various theories and evidence contributing to our understanding of this celestial body. At the heart of this investigation are three key areas of study: geological and geochemical data, astronomical observations and simulations, and comparative planetary science. Geological and geochemical data provide insights into the Moon's composition and structure, offering clues about its origins through the analysis of lunar samples and terrestrial analogs. Astronomical observations and simulations help scientists reconstruct the early solar system, modeling scenarios that could have led to the Moon's creation. Meanwhile, comparative planetary science allows researchers to draw parallels between the Moon and other celestial bodies, shedding light on common formation mechanisms. By integrating these diverse lines of evidence, scientists aim to piece together a comprehensive narrative of the Moon's genesis. This article delves into these critical areas of research, ultimately leading to a deeper understanding of the Moon's formation.
Geological and Geochemical Data
Geological and geochemical data play a pivotal role in understanding the formation and evolution of celestial bodies, including the Moon. These data sets are crucial for reconstructing the Moon's history, from its initial formation to its current state. Geological data, derived from lunar samples and remote sensing, provide insights into the Moon's surface and subsurface structures. For instance, the Apollo missions brought back over 800 pounds of lunar rocks and soil, which have been extensively analyzed to determine their mineral composition, age, and origin. These samples reveal that the Moon is primarily composed of silicate minerals such as feldspar, pyroxene, and olivine, indicating a magmatic differentiation process early in its history. Geochemical analysis of these samples has also shed light on the Moon's isotopic signature. The similarity in isotopic ratios of elements like oxygen between Earth and Moon suggests a common origin or a significant exchange of material during their early stages. This evidence supports the giant impact hypothesis, which posits that the Moon formed from debris left over after a massive collision between Earth and a Mars-sized object called Theia. Geochemical data further indicate that this collision would have caused extensive melting and differentiation, resulting in the Moon's distinct crust, mantle, and core. Moreover, geochemical studies have helped in understanding the Moon's thermal evolution. The presence of certain minerals and the absence of others provide clues about the Moon's cooling history. For example, the lack of water and volatile compounds in lunar samples suggests that the Moon experienced a high-temperature environment during its early stages, which would have driven off these volatiles. This thermal history is consistent with models that propose a large-scale melting event following the giant impact. Remote sensing technologies, including orbital missions like NASA's Lunar Reconnaissance Orbiter, have complemented ground-based data by providing detailed topographic maps and compositional analyses of the lunar surface. These data help in identifying different geological units such as maria (large basaltic plains) and highlands (ancient crustal rocks), which are critical for reconstructing the Moon's geological timeline. In summary, geological and geochemical data form the backbone of scientific evidence supporting theories about the Moon's origin and evolution. By integrating these data with theoretical models, scientists can reconstruct a comprehensive narrative of how the Moon came to be and how it has changed over billions of years. This interdisciplinary approach not only enhances our understanding of the Moon but also provides valuable insights into the broader context of planetary formation within our solar system.
Astronomical Observations and Simulations
Astronomical observations and simulations are cornerstone components in the pursuit of understanding celestial phenomena, including the formation and evolution of our solar system. These tools allow scientists to gather empirical data and model complex processes, thereby bridging the gap between theoretical frameworks and real-world evidence. Observations involve the systematic collection of data from various astronomical objects and events, such as planetary motions, stellar life cycles, and cosmic microwave background radiation. Telescopes, both ground-based and space-based, play a crucial role in this endeavor by providing high-resolution images and spectra that reveal detailed information about the physical properties of celestial bodies. Simulations, on the other hand, involve the use of computational models to replicate these phenomena under controlled conditions. By inputting known physical laws and initial conditions into sophisticated algorithms, researchers can predict how systems might evolve over time. For instance, simulations of planetary formation help explain how planets like Earth could have formed from a disk of gas and dust surrounding the Sun. Similarly, simulations of stellar evolution provide insights into how stars like our Sun undergo changes throughout their lifetimes. The synergy between observations and simulations is essential for validating scientific theories. For example, observations of the cosmic microwave background radiation provided strong evidence for the Big Bang theory, while simulations helped predict what this radiation should look like if the universe began in such an event. In another context, observations of exoplanet transits are complemented by simulations that model the atmospheric properties of these distant worlds, allowing scientists to infer their potential habitability. Moreover, advancements in technology have significantly enhanced both observational capabilities and simulation accuracy. Next-generation telescopes like the James Webb Space Telescope and the Square Kilometre Array will offer unprecedented resolution and sensitivity, enabling deeper insights into the universe's earliest moments and most distant reaches. Concurrently, improvements in computational power and algorithmic efficiency allow for more detailed and realistic simulations that can better capture the complexities of astrophysical processes. In summary, astronomical observations and simulations form a robust framework for scientific inquiry in astronomy. By combining empirical data with predictive models, researchers can test hypotheses, refine theories, and gain a deeper understanding of the cosmos. This interplay between observation and simulation not only strengthens our current knowledge but also paves the way for future discoveries that will continue to unravel the mysteries of the universe.
Comparative Planetary Science
Comparative planetary science is a dynamic field that seeks to understand the formation, evolution, and characteristics of celestial bodies within our solar system and beyond. By comparing the diverse planets, moons, asteroids, and comets, scientists can uncover universal principles and unique features that shed light on the complex history of our cosmos. This interdisciplinary approach integrates insights from geology, astronomy, atmospheric science, and biology to provide a comprehensive view of planetary systems. One of the key areas of focus in comparative planetary science is the study of planetary differentiation. For instance, Earth's iron-rich core and silicate mantle are contrasted with Mars' partially differentiated interior, highlighting differences in thermal evolution and tectonic activity. The Moon's own differentiation is particularly intriguing; its iron-depleted composition suggests a violent collision early in its history, possibly involving a Mars-sized object known as Theia. This collision, often referred to as the Giant Impact Hypothesis, is supported by similarities in Earth and Moon isotopic signatures and the Moon's relatively small iron core. Atmospheric studies also play a crucial role in comparative planetary science. The atmospheres of Venus and Earth are often compared due to their similar size and proximity to the Sun but vastly different climates. Venus's thick atmosphere traps heat in a runaway greenhouse effect, resulting in surface temperatures that can melt lead. In contrast, Earth's atmosphere supports life through a delicate balance of gases. Mars' thin atmosphere offers insights into how planetary environments can change over time; its current state suggests a past with flowing water and possibly even life. The moons of other planets provide additional avenues for comparison. Jupiter's moon Europa and Saturn's moon Enceladus are of particular interest due to their subsurface oceans, which are thought to be in contact with rock and could potentially harbor life. These findings underscore the importance of liquid water in astrobiology and highlight the diversity of habitable environments beyond Earth. Theoretical models and scientific evidence from comparative planetary science also inform our understanding of planetary formation theories such as the Nebular Hypothesis and the Grand Tack Hypothesis. These theories propose that planets form from disks of gas and dust surrounding young stars and that their orbits can be influenced by gravitational interactions with other bodies. By comparing the orbital patterns and compositions of planets in our solar system with those observed in exoplanetary systems, scientists can refine these theories and gain a deeper understanding of how planetary systems evolve over billions of years. In summary, comparative planetary science leverages a broad range of scientific disciplines to elucidate the complexities of celestial bodies. Through detailed comparisons and analyses, researchers can reconstruct the histories of planets and moons, identify commonalities and differences, and ultimately enhance our understanding of the universe's formation and evolution. This field not only enriches our knowledge of the solar system but also provides critical context for ongoing searches for life beyond Earth.
Timeline and Key Events in Lunar History
The Moon, Earth's faithful companion in the vast expanse of space, has a rich and complex history that spans billions of years. Understanding this history is crucial for grasping the intricate processes that have shaped our celestial neighbor. This article delves into the timeline and key events in lunar history, exploring three pivotal phases: **Formation and Early Cooling**, which examines the Moon's origins and its initial stages of development; **Moon's Surface Evolution**, which details the significant transformations that have occurred on the lunar surface over time; and **Recent Geological Activity**, which highlights the more recent changes and ongoing processes that continue to shape the Moon today. By examining these critical periods, we can gain a deeper understanding of how the Moon came to be what it is today, ultimately leading us to a comprehensive appreciation of its formation and evolution. Transitioning seamlessly into **Understanding the Moon's Formation**, this journey through lunar history will provide readers with a robust foundation for appreciating the Moon's place in our solar system.
Formation and Early Cooling
The formation and early cooling of the Moon are pivotal events in lunar history, offering insights into the Moon's origins and evolution. The most widely accepted theory is the giant impact hypothesis, which suggests that the Moon formed approximately 4.5 billion years ago from debris left over after a massive collision between Earth and a Mars-sized object known as Theia. This cataclysmic event is believed to have ejected a significant portion of Earth's mantle and crust into space, where it coalesced to form the Moon. Following its formation, the Moon underwent a period of intense heating due to the energy released from the impact and subsequent gravitational differentiation. This process led to the melting of a large portion of the Moon's interior, creating a global magma ocean. As this magma ocean cooled, it solidified into distinct layers, with heavier elements like iron sinking to the core and lighter materials rising to form the crust. The early cooling phase was marked by extensive volcanic activity, as evidenced by the widespread presence of dark basaltic maria (seas) on the Moon's surface. These maria are composed of solidified lava flows that erupted from the Moon's interior during this period of volcanic turmoil. The cooling process also facilitated the formation of anorthositic crust, which is rich in calcium and aluminum silicates and forms much of the Moon's lighter-colored highlands. As the Moon continued to cool, it entered a phase known as "lunar differentiation," where heavier elements separated from lighter ones, leading to the development of a small iron-rich core surrounded by a partially molten mantle. This differentiation process played a crucial role in shaping the Moon's internal structure and influencing its subsequent geological evolution. The early cooling phase was also characterized by a significant reduction in the Moon's internal heat budget, which gradually diminished volcanic activity and tectonic processes. By about 3.8 billion years ago, the Moon had cooled sufficiently to allow for the formation of a stable crust, marking the end of its most intense geological activity. Understanding these early stages in lunar history provides valuable context for interpreting subsequent events in the Moon's timeline, such as the impact cratering that shaped its surface and the eventual cessation of major geological processes. The formation and early cooling of the Moon set the stage for its long-term evolution, influencing its composition, structure, and surface features that we observe today.
Moon's Surface Evolution
The Moon's surface evolution is a complex and fascinating narrative that spans over 4.5 billion years, intricately woven with key events that have shaped its current appearance. The journey begins with the Moon's formation, believed to have occurred when a massive object collided with Earth, causing debris to coalesce into our satellite. This early period, known as the Hadean Eon, was marked by intense volcanic and tectonic activity as the Moon cooled and solidified. As it entered the Nectarian Period, around 3.9 billion years ago, the Moon experienced a series of massive impacts that formed the large dark basaltic plains known as "maria," which are visible from Earth. These impacts were so powerful that they excavated deep craters and deposited layers of molten rock that eventually solidified into the maria we see today. The Imbrian Period, which followed, saw the formation of some of the Moon's most prominent features, including the Mare Imbrium and Mare Serenitatis. This era was characterized by a significant decrease in impact events but an increase in volcanic activity, leading to the filling of impact basins with dark basaltic lava flows. The subsequent Eratosthenian Period marked a transition towards a more geologically quiet phase, with fewer impacts and volcanic eruptions. However, this period also saw the formation of smaller, more localized volcanic features such as domes and cones. In the Copernican Period, which extends from about 1.2 billion years ago to the present, the Moon's surface has been relatively stable, with minimal tectonic or volcanic activity. This era has been dominated by smaller impact events that have created the numerous craters seen across the lunar surface. The most recent impacts have left behind bright ejecta rays that are visible against the darker background of older surfaces. Throughout its evolution, the Moon's surface has also been influenced by solar wind and meteoritic bombardment, which have contributed to its weathered appearance. The lunar regolith, or soil, has been formed through the continuous breakdown of rocks by these external forces. This regolith is crucial for understanding the Moon's history as it contains a record of past events in the form of impact debris and solar wind particles. In summary, the Moon's surface evolution is a testament to its dynamic and violent past, shaped by colossal impacts, volcanic eruptions, and external influences. Each period in lunar history has left its mark, creating the diverse and intriguing landscape we observe today. This timeline of key events provides a comprehensive framework for understanding how our Moon transformed from a molten, chaotic body into the serene yet rugged satellite we admire in the night sky.
Recent Geological Activity
Recent geological activity on the Moon, though significantly less intense than its early history, continues to shape our understanding of lunar evolution. Despite the Moon's surface appearing static, there are several indicators of ongoing geological processes. One key area of interest is the presence of "moonquakes," which were first detected by seismometers left on the Moon's surface during the Apollo missions. These quakes, often caused by tectonic activity or the cooling and contraction of the Moon's interior, provide valuable insights into the Moon's internal structure and composition. Additionally, observations from orbiting spacecraft have revealed evidence of recent volcanic activity, such as the existence of "dark spots" or "lunar domes," which are thought to be volcanic features formed by the eruption of lava flows. These features suggest that volcanic processes may have continued on the Moon until relatively recently, possibly within the last billion years. The study of lunar regolith also offers clues about recent geological activity. The regolith, a layer of loose, fragmented rock that covers the Moon's surface, is constantly being reshaped by meteorite impacts and solar wind. This dynamic process indicates that even in the absence of tectonic or volcanic activity, the Moon's surface is still undergoing significant changes. Furthermore, NASA's Lunar Reconnaissance Orbiter has provided high-resolution imagery that highlights recent impact craters and other surface features, demonstrating that the Moon remains geologically active in a subtle yet persistent manner. Understanding these recent geological activities is crucial for piecing together the Moon's timeline and key events in its history. By analyzing these phenomena, scientists can better reconstruct the Moon's thermal evolution, its internal dynamics, and how these factors have influenced its surface over billions of years. This knowledge not only enhances our comprehension of lunar history but also provides valuable context for future lunar missions aimed at exploring and utilizing the Moon's resources. In summary, while the Moon's geological activity is far less vigorous than in its formative stages, ongoing processes such as moonquakes, volcanic remnants, and regolith dynamics underscore that the Moon remains an evolving body. These recent activities serve as a bridge between the Moon's ancient past and its present state, offering scientists a richer understanding of how this celestial body has transformed over time. As we continue to explore and study the Moon, these insights will be essential for mapping out its full timeline and key events in its history.