When We Collided
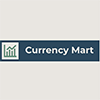
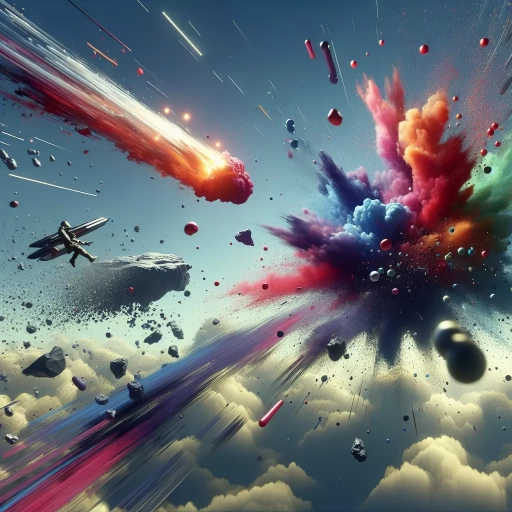
In the vast expanse of the universe, collisions have been a pivotal force shaping the cosmos as we know it. From the grand scale of galaxy mergers to the microscopic impacts of subatomic particles, these events have left indelible marks on our understanding of space and time. This article delves into the multifaceted nature of collisions, exploring three critical dimensions: **Understanding the Concept of Time and Collision**, **Scientific Theories and Evidence of Collisions**, and **Implications and Consequences of Collisions**. By examining how our perception of time intersects with the phenomenon of collision, we uncover the intricate dance between temporal progression and physical impact. We will then delve into the scientific theories and empirical evidence that underpin our knowledge of collisions, highlighting key discoveries and observations. Finally, we will discuss the profound implications and consequences that arise from these events, influencing everything from the formation of celestial bodies to the potential for catastrophic outcomes. To begin this journey, let us first **Understand the Concept of Time and Collision**, a foundational element that sets the stage for a deeper exploration of this complex and fascinating topic.
Understanding the Concept of Time and Collision
Understanding the concept of time and collision is a multifaceted endeavor that delves into the very fabric of our universe. At its core, this exploration requires a deep dive into the scientific definition of time, which underpins our comprehension of temporal dynamics. In this article, we will first **define time in scientific terms**, examining how physicists and mathematicians quantify and study this fundamental dimension. Next, we will delve into **theoretical frameworks for understanding collisions**, exploring the principles of mechanics and relativity that govern interactions between objects. Additionally, we will provide **historical perspectives on time and space**, tracing the evolution of thought from ancient philosophers to modern theorists. By integrating these perspectives, we aim to provide a comprehensive understanding of how time and collision intersect, shedding light on the intricate dance between these two concepts. Ultimately, this article seeks to illuminate the intricate relationship between time and collision, offering readers a nuanced grasp of these interconnected phenomena.
Defining Time in Scientific Terms
Defining time in scientific terms is a complex yet fascinating endeavor that delves into the very fabric of our universe. At its core, time is a dimension that allows us to measure the duration between events and the sequence in which they occur. In physics, time is often described as the fourth dimension, complementing the three spatial dimensions. This concept is central to Einstein's theory of relativity, where time is not an absolute quantity but rather a relative one, influenced by gravity and motion. According to special relativity, time can appear to slow down or speed up depending on an object's velocity relative to an observer, a phenomenon known as time dilation. General relativity further expands this idea by introducing gravitational time dilation, where the presence of mass warps spacetime, causing time to pass differently at various gravitational potentials. The scientific definition of time also involves understanding its quantification. The second, defined as the duration of 9,192,631,770 periods of the radiation corresponding to the transition between the two hyperfine levels of the ground state of the caesium-133 atom, serves as the fundamental unit of time in the International System of Units (SI). This precise measurement allows for the synchronization of clocks across the globe, ensuring a unified understanding of time. Moreover, the concept of time is deeply intertwined with the laws of thermodynamics, particularly the second law, which states that entropy always increases over time in a closed system. This directional arrow of time explains why we experience events in a linear fashion—from past to present to future—rather than in reverse. In the context of collisions, understanding time becomes crucial. When objects collide, their trajectories and interactions are governed by the laws of physics, which are inherently time-dependent. The timing and sequence of these interactions determine the outcomes of collisions, whether it be the conservation of momentum and energy or the deformation and damage to the colliding objects. Thus, defining time scientifically not only provides a framework for understanding the universe but also enables precise predictions and analyses of physical phenomena, including the intricate dynamics of collisions. By grasping the scientific definition of time, we gain a deeper insight into the mechanisms that govern our world and the events that shape it.
Theoretical Frameworks for Understanding Collisions
Understanding collisions requires a robust theoretical framework that encapsulates the intricate dynamics involved. At the heart of this understanding lies the concept of time, which is pivotal in defining the sequence and duration of events. Theoretical frameworks such as classical mechanics, quantum mechanics, and relativity provide distinct yet complementary perspectives on collisions. Classical mechanics, as formulated by Sir Isaac Newton, offers a foundational framework for understanding macroscopic collisions. Newton's laws of motion and the law of universal gravitation describe how objects interact and respond to forces. In this context, collisions are analyzed using concepts like momentum, energy conservation, and impulse. For instance, the principle of conservation of momentum states that the total momentum before a collision is equal to the total momentum after the collision, providing a quantitative tool to predict outcomes. However, as we delve into the microscopic realm, classical mechanics becomes insufficient. Quantum mechanics, developed by pioneers such as Max Planck and Niels Bohr, introduces probabilistic principles that govern particle interactions. Here, collisions are described using wave functions and probability amplitudes. Quantum field theory further refines these concepts by treating particles as excitations of underlying fields, enabling the study of high-energy collisions where particle creation and annihilation occur. Relativity, particularly special relativity by Albert Einstein, adds another layer of complexity by integrating time dilation and length contraction into collision dynamics. This framework is crucial for understanding high-speed collisions where relativistic effects become significant. For example, in particle accelerators, collisions at nearly the speed of light necessitate relativistic corrections to accurately predict particle trajectories and interaction energies. Moreover, modern theoretical frameworks like quantum field theory and string theory attempt to unify these disparate approaches. Quantum field theory merges quantum mechanics with special relativity, providing a consistent description of particle interactions at all scales. String theory, though still speculative, posits that fundamental particles are not point-like but tiny vibrating strings, offering a potential resolution to the long-standing problem of reconciling quantum mechanics with general relativity. In summary, understanding collisions requires an interdisciplinary approach that leverages classical mechanics for macroscopic phenomena, quantum mechanics for microscopic interactions, and relativity for high-speed dynamics. These theoretical frameworks collectively provide a comprehensive toolkit for analyzing and predicting collision outcomes across various scales and regimes, thereby enriching our comprehension of time and its role in defining these events. By integrating these perspectives, we can gain a deeper insight into the intricate dance of particles and objects as they collide, reflecting the intricate interplay between time, space, and matter.
Historical Perspectives on Time and Space
Historical perspectives on time and space have evolved significantly over centuries, reflecting the intellectual and technological advancements of their respective eras. In ancient civilizations, such as those in Greece and China, time was often seen as cyclical, with events repeating themselves in an eternal cycle. This view was deeply intertwined with religious and mythological beliefs, where the passage of time was tied to the actions of gods and the natural world. For instance, the ancient Greeks believed in the concept of eternal return, where all events would recur infinitely. The advent of Christianity introduced a linear concept of time, where history unfolded in a straight line from creation to the apocalypse. This linear perspective dominated Western thought for centuries and influenced how people perceived their place within the timeline of human history. The Middle Ages saw the development of elaborate systems for measuring time, including the use of sundials and water clocks, which further solidified the notion of time as a quantifiable and divisible entity. The Renaissance and Enlightenment periods brought about a significant shift with the rise of scientific inquiry. Sir Isaac Newton's laws of motion and universal gravitation introduced a mechanistic view of time and space, where both were seen as absolute and unchanging. According to Newton, time flowed uniformly and independently of human perception, much like a clock ticking away in the background. However, this Newtonian view was challenged by Albert Einstein's theory of relativity in the early 20th century. Einstein's work revealed that time and space are not fixed but are relative to the observer's frame of reference. Time dilation and length contraction became key concepts, showing how time and space can be affected by gravity and motion. This revolutionary idea transformed our understanding of the cosmos and our place within it. In contemporary times, quantum mechanics has further complicated our understanding of time and space. Theories such as quantum gravity and certain interpretations of quantum mechanics suggest that time may not be fundamental but rather an emergent property of the universe. These modern perspectives highlight the dynamic nature of our understanding of time and space, which continues to evolve as new discoveries are made. Understanding these historical perspectives is crucial for grasping the concept of time and collision. By recognizing how different cultures and scientists have perceived time and space over history, we can better appreciate the complexities involved in understanding collisions—whether they be cosmic events like asteroid impacts or subatomic interactions within particle accelerators. Each historical perspective adds a layer to our comprehension, allowing us to see how our current understanding is built upon a rich tapestry of past insights and discoveries.
Scientific Theories and Evidence of Collisions
Scientific theories and evidence of collisions span a vast spectrum, from the cosmic to the quantum, each offering unique insights into the fundamental nature of our universe. At the cosmological scale, the Big Bang Theory provides a framework for understanding the origins of the universe, positing that all matter began from a singular point and has been expanding ever since. This theory is supported by a wealth of observational evidence, including the cosmic microwave background radiation and the abundance of light elements. On a more localized level, astronomical observations reveal frequent galaxy collisions, which reshape the structure of our cosmos and provide valuable data on gravitational interactions. Delving into the microscopic realm, quantum mechanics elucidates particle collisions, where subatomic particles interact and transform, governed by probabilistic laws that underpin much of modern physics. These diverse perspectives on collisions collectively enrich our understanding of the universe's dynamics. By exploring these theories and observations, we can transition seamlessly to a deeper comprehension of the intricate relationship between time and collision, revealing how these phenomena are intertwined in the fabric of space and time.
Cosmological Models: Big Bang Theory
The Big Bang Theory is the cornerstone of modern cosmology, providing a comprehensive framework for understanding the origin and evolution of the universe. This theory posits that approximately 13.8 billion years ago, the universe began as an infinitely hot and dense point known as a singularity. From this singularity, the universe expanded rapidly in an event known as the Big Bang. This expansion continues to this day, with galaxies moving away from each other at speeds proportional to their distance. Key evidence supporting the Big Bang Theory includes the cosmic microwave background radiation (CMB), which is the residual heat from the initial explosion. Discovered in 1964 by Arno Penzias and Robert Wilson, the CMB is a uniform radiation that fills the universe, consistent with the predictions of the Big Bang model. Another crucial piece of evidence is the abundance of light elements such as hydrogen, helium, and lithium, which are thought to have been formed during the first few minutes after the Big Bang in a process known as Big Bang nucleosynthesis. The large-scale structure of the universe also supports the Big Bang Theory. Galaxies and galaxy clusters are observed to be distributed in a web-like pattern, which can be explained by the gravitational collapse of tiny fluctuations in the density of the universe during its early stages. These fluctuations are evident in the CMB and have been mapped in detail by satellites such as COBE, WMAP, and Planck. Furthermore, the redshift of light from distant galaxies, first observed by Edwin Hubble in 1929, indicates that these galaxies are moving away from us. This observation led to Hubble's Law, which states that the velocity of a galaxy is directly proportional to its distance from us. This relationship is a strong indicator of the expanding universe predicted by the Big Bang Theory. In addition to these observational evidences, theoretical models such as inflationary cosmology have been developed to explain certain features of the universe that are not fully addressed by the standard Big Bang model. Inflation suggests that the universe underwent a rapid expansion in its very early stages, smoothing out any irregularities and explaining why the universe appears so homogeneous on large scales. In conclusion, the Big Bang Theory is well-supported by a wealth of observational and theoretical evidence. It provides a coherent explanation for the origins and evolution of our cosmos, from the formation of light elements to the large-scale structure of galaxies. As part of broader scientific theories and evidence of collisions within our universe, understanding the Big Bang Theory offers profound insights into how cosmic events shape our understanding of space and time.
Astronomical Observations: Galaxy Collisions
Astronomical observations of galaxy collisions offer a fascinating glimpse into the dynamic and ever-evolving universe. These cosmic events, where galaxies merge or collide, are pivotal in understanding the formation and evolution of galaxies over billions of years. Observations from advanced telescopes such as the Hubble Space Telescope and the Atacama Large Millimeter/submillimeter Array (ALMA) have provided detailed insights into these interactions. For instance, the iconic collision between the Milky Way and Andromeda galaxies, predicted to occur in about 4.5 billion years, is already being studied through simulations and observations of their current trajectories. Galaxy collisions trigger a cascade of astrophysical processes. The gravitational interaction between colliding galaxies can lead to the formation of new stars as gas clouds are compressed and shocked, initiating starbursts. This is evident in observations of galaxies like the Antennae Galaxies (NGC 4038/4039), where intense star formation is observed along the collision interface. Additionally, these events can result in the ejection of gas and dust into intergalactic space, influencing the chemical composition of the surrounding medium. The evidence for galaxy collisions is multifaceted. Astronomers observe distorted galaxy shapes, tidal tails of stars and gas, and the presence of young stellar populations indicative of recent starbursts. Spectroscopic data reveal the kinematic signatures of merging galaxies, such as unusual velocity distributions and emission lines characteristic of shocked gas. Furthermore, gravitational lensing effects around massive galaxy clusters provide indirect evidence of ongoing mergers by bending light around these cosmic structures. Theoretical models supported by these observations suggest that galaxy collisions are a common occurrence throughout cosmic history. Simulations based on the Lambda-CDM model of the universe predict frequent mergers during the early universe, contributing significantly to the growth and morphological diversity of galaxies we see today. These simulations align well with observational data, reinforcing our understanding that galaxy collisions are a fundamental aspect of galaxy evolution. In conclusion, astronomical observations of galaxy collisions not only captivate with their visual beauty but also provide crucial evidence for scientific theories about the dynamic evolution of galaxies. By studying these events, astronomers gain insights into the mechanisms driving star formation, gas dynamics, and the overall structure of the universe. As observational techniques continue to advance, our understanding of these cosmic interactions will become even more refined, offering deeper insights into the history and future of our universe.
Quantum Mechanics: Particle Collisions
Quantum Mechanics: Particle Collisions is a cornerstone of modern physics, offering profound insights into the behavior of matter at the smallest scales. This field delves into the interactions between subatomic particles, such as electrons, protons, and photons, which are fundamental to understanding the structure and dynamics of atoms and molecules. In particle collisions, scientists observe how these tiny entities interact and transform, often resulting in the creation of new particles or the emission of energy. The principles of quantum mechanics govern these interactions, where particles exhibit wave-like behavior and probabilistic outcomes. The Heisenberg Uncertainty Principle, for instance, dictates that certain properties of particles, like position and momentum, cannot be precisely known simultaneously. This inherent uncertainty is crucial in predicting the outcomes of particle collisions, where the probability of different outcomes is calculated using wave functions and Schrödinger's equation. Experimental evidence from high-energy particle accelerators, such as the Large Hadron Collider (LHC), has been instrumental in validating quantum mechanical predictions. These accelerators collide particles at nearly the speed of light, recreating conditions similar to those in the early universe. The detection of the Higgs boson in 2012, a particle predicted by the Standard Model of particle physics, is a prime example of how experimental data supports theoretical frameworks. The Higgs boson's discovery confirmed the existence of the Higgs field, which explains how particles acquire mass. Furthermore, quantum field theory (QFT) provides a comprehensive framework for understanding particle interactions. QFT describes particles as excitations of underlying fields that permeate space and time. This theory has been remarkably successful in predicting cross-sections for various collision processes and has been validated through numerous experiments. The study of particle collisions also sheds light on fundamental forces such as electromagnetism and the strong and weak nuclear forces. For example, electron-positron annihilation experiments have provided detailed insights into electromagnetic interactions, while proton-proton collisions at the LHC have helped elucidate the strong force's role in holding quarks together within protons and neutrons. In addition to advancing our understanding of fundamental physics, research in particle collisions has practical implications. It drives technological innovation, such as the development of advanced detectors and computational tools, which have spin-off benefits in fields like medicine and materials science. The pursuit of understanding particle collisions continues to push the boundaries of human knowledge, offering a glimpse into the intricate and fascinating world governed by quantum mechanics. As scientists continue to probe deeper into the nature of matter and energy through these collisions, they uncover new mysteries and opportunities for discovery that enrich our understanding of the universe.
Implications and Consequences of Collisions
Collisions, whether between celestial bodies or other entities, have profound implications that resonate across various domains of our universe and human experience. The consequences of these events are multifaceted, influencing the very fabric of planetary formation and evolution, shaping the trajectory of human history and civilization, and posing significant risks for our future. In the realm of planetary formation, collisions play a crucial role in shaping the structure and composition of planets, moons, and asteroids. On Earth, historical collisions have left indelible marks on human history, from the extinction of species to the creation of impact craters that serve as geological time capsules. Looking ahead, future predictions highlight potential risks associated with asteroid impacts and other cosmic collisions, underscoring the need for vigilant monitoring and preparedness. This article delves into these critical aspects—Impact on Planetary Formation and Evolution, Effects on Human History and Civilization, and Future Predictions and Potential Risks—to provide a comprehensive understanding of the far-reaching consequences of collisions. By exploring these dimensions, we gain a deeper insight into the dynamic nature of our universe and the interconnectedness of time and collision. Understanding the Concept of Time and Collision becomes essential as we navigate the complexities of a universe where impacts are both destructive and transformative.
Impact on Planetary Formation and Evolution
The impact of collisions on planetary formation and evolution is profound and multifaceted. During the early stages of solar system development, frequent collisions between protoplanets and other celestial bodies played a crucial role in shaping the final architecture of our planetary system. These impacts could either lead to the accretion of material, contributing to the growth of larger bodies, or result in catastrophic disruptions that fragmented and dispersed material into space. For instance, the Moon is believed to have formed from debris left over after a massive collision between Earth and a Mars-sized object known as Theia. This event not only formed the Moon but also significantly altered Earth's rotation rate and possibly its magnetic field. Collisions also influenced the distribution of elements within planets. The delivery of volatile compounds such as water and organic molecules via comets and asteroids during late-stage bombardment periods is thought to have contributed to the habitability of Earth. Conversely, high-energy impacts could strip away atmospheric gases, making it difficult for some planets to retain an atmosphere conducive to life. The frequency and intensity of collisions during planetary formation can also determine the final composition and structure of planets. For example, Mercury's unusually large iron core relative to its mantle is hypothesized to be the result of a giant impact that stripped away much of its silicate crust. Similarly, Venus's slow rotation rate might be a consequence of gravitational interactions or impacts that altered its angular momentum. Furthermore, collisions have shaped the orbital dynamics of our solar system. The Nice Model suggests that Jupiter's migration due to gravitational interactions with the solar nebula led to a period of intense bombardment known as the Late Heavy Bombardment, which reshaped the surfaces of inner planets like Earth and Mars around 3.8 billion years ago. In summary, collisions have been instrumental in sculpting the diverse range of planets we observe today. They have influenced planetary size, composition, atmospheric retention, and orbital paths, ultimately contributing to the complex and varied landscape of our solar system. Understanding these impacts provides valuable insights into how planetary systems form and evolve over billions of years, highlighting the dynamic and often violent history that underpins the tranquility we see today.
Effects on Human History and Civilization
The impact of collisions on human history and civilization has been profound and multifaceted. From the earliest recorded events to modern times, collisions—whether they be asteroid impacts, wars, or cultural exchanges—have shaped the trajectory of human societies in significant ways. One of the most dramatic examples is the asteroid impact that occurred around 66 million years ago, which led to the extinction of the dinosaurs and paved the way for the rise of mammals and eventually, humans. This event underscores how external forces can drastically alter the course of life on Earth. In historical terms, collisions between different cultures and civilizations have often led to periods of significant change and innovation. The collision of ancient Greek and Roman cultures, for instance, resulted in a rich exchange of ideas that laid the foundation for Western philosophy, art, and governance. Similarly, the Mongol invasions of Europe in the 13th century facilitated the transfer of knowledge from East Asia to Europe, contributing to the Renaissance and the Age of Exploration. These cultural collisions not only expanded geographical horizons but also enriched intellectual and artistic landscapes. Wars and conflicts have also been a form of collision that has reshaped human history. The World Wars, for example, led to the reorganization of global politics, the establishment of the United Nations, and a new era of international relations. These conflicts also spurred technological advancements, such as radar technology and nuclear energy, which have had lasting impacts on modern society. Moreover, economic collisions—such as trade wars and financial crises—have influenced economic systems and global stability. The Great Depression of the 1930s, triggered by a combination of economic policies and market failures, led to widespread suffering but also prompted the development of new economic theories and regulatory frameworks that continue to shape global economic policies today. In addition to these macro-level impacts, personal collisions—encounters between individuals from different backgrounds—have played a crucial role in shaping individual lives and societal norms. The Civil Rights Movement in the United States, for example, was driven by collisions between activists and the status quo, leading to significant legal and social changes that continue to influence contemporary society. In conclusion, collisions have been a driving force behind many of the pivotal moments in human history. Whether they involve celestial bodies, cultures, nations, or individuals, these interactions have consistently led to transformative outcomes that have reshaped the world we live in today. Understanding these collisions provides valuable insights into how human civilization has evolved and how it might continue to evolve in response to future challenges and opportunities.
Future Predictions and Potential Risks
As we navigate the complexities of a rapidly evolving world, future predictions and potential risks become increasingly critical for informed decision-making. The implications and consequences of collisions, whether they be technological, societal, or environmental, demand careful consideration. In the realm of technology, the integration of artificial intelligence and machine learning could lead to unprecedented advancements but also poses significant risks. For instance, the misuse of AI could result in job displacement on a massive scale, exacerbating economic inequality and social unrest. Moreover, the potential for AI systems to malfunction or be exploited for malicious purposes underscores the need for robust ethical frameworks and regulatory oversight. In the societal sphere, demographic shifts and urbanization trends are likely to reshape global dynamics. The aging population in many developed countries could strain healthcare systems and pension funds, while urbanization may lead to increased resource competition and environmental degradation. Climate change, a pressing environmental issue, is expected to intensify natural disasters and alter ecosystems, displacing communities and disrupting global food supplies. The consequences of these changes will be far-reaching, necessitating proactive policies to mitigate their impact. Economically, the rise of digital currencies and decentralized finance (DeFi) promises greater financial inclusion but also introduces new vulnerabilities. Cybersecurity threats could compromise entire financial systems, leading to economic instability and widespread financial loss. Additionally, geopolitical tensions may escalate as nations vie for dominance in emerging technologies, potentially triggering conflicts that could have devastating global repercussions. To navigate these challenges effectively, it is essential to foster a culture of foresight and resilience. This involves investing in research and development to address emerging risks, implementing adaptive governance structures, and promoting international cooperation to tackle shared challenges. Public awareness campaigns can also play a crucial role in preparing societies for the potential consequences of these collisions, encouraging proactive measures such as disaster preparedness and sustainable practices. Ultimately, the future is inherently uncertain, but by acknowledging potential risks and taking proactive steps to mitigate them, we can better prepare ourselves for the implications and consequences of collisions across various domains. This proactive approach not only safeguards our well-being but also positions us to capitalize on the opportunities that arise from these intersections, driving innovation and progress in a rapidly changing world.