When Will The Next Ice Age Happen
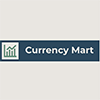
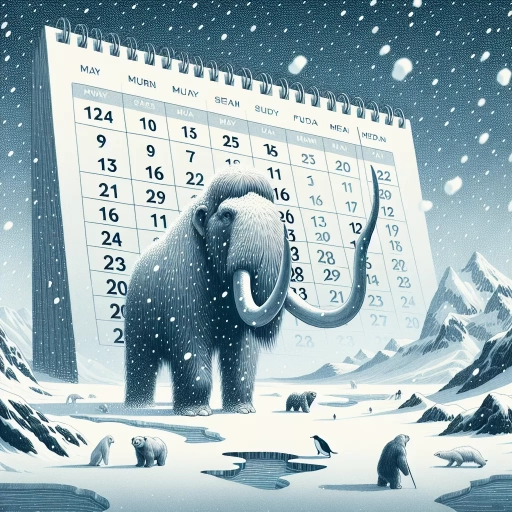
The prospect of another ice age has long fascinated scientists and the general public alike, sparking both curiosity and concern about the future of our planet. As we navigate the complexities of climate change, understanding when the next ice age might occur is crucial for predicting and preparing for potential environmental shifts. This article delves into three key areas to shed light on this question: **Understanding the Ice Age Cycle**, which explores the historical patterns and natural processes that govern ice ages; **Current Climate Trends and Predictions**, which examines the latest data and forecasts from climate science; and **Factors Influencing the Onset of the Next Ice Age**, which considers the various elements that could hasten or delay this event. By examining these aspects, we can gain a comprehensive insight into the likelihood and timing of the next ice age. Let us begin by **Understanding the Ice Age Cycle**, a foundational step in grasping the broader context of Earth's climatic history.
Understanding the Ice Age Cycle
The Earth's climate has oscillated between periods of significant glaciation and warmer interglacial periods, a phenomenon known as the Ice Age cycle. This cyclical pattern has shaped our planet's history, influencing ecosystems, human migration, and the evolution of life. To understand the Ice Age cycle, it is crucial to delve into its historical patterns, which reveal recurring episodes of ice sheet expansion and contraction. Additionally, orbital variations play a pivotal role in these cycles, as changes in Earth's orbit around the Sun affect the distribution of solar energy. Furthermore, volcanic eruptions have a profound impact on global climate, often triggering or exacerbating ice age conditions. By examining these historical patterns, the role of orbital variations, and the impact of volcanic eruptions, we can gain a comprehensive understanding of the Ice Age cycle and its profound implications for our planet's climate and life. This article will explore these key factors in depth, providing a detailed insight into the complex dynamics that drive the Ice Age cycle.
Historical Patterns of Ice Ages
Historical patterns of ice ages are crucial for understanding the cyclical nature of Earth's climate. Over the past 2.5 million years, Earth has experienced repeated periods of significant glaciation, known as ice ages, interspersed with warmer interglacial periods. These cycles are primarily driven by variations in Earth's orbital parameters, such as changes in the tilt of its axis, the shape of its orbit around the Sun, and the precession of its equinoxes. These orbital variations, described by Milankovitch cycles, affect the distribution of solar energy received by Earth, leading to fluctuations in global temperatures. During ice ages, large portions of the Northern Hemisphere are covered by massive ice sheets, such as those that once spanned North America and Eurasia. These ice sheets reflect sunlight back into space, further cooling the planet and creating a feedback loop that enhances glaciation. Conversely, during interglacial periods like the current Holocene epoch, these ice sheets retreat, allowing more solar radiation to be absorbed and warming the climate. One of the most well-documented ice ages is the Pleistocene glaciation, which lasted from approximately 110,000 to 10,000 years ago. This period saw multiple glacial maxima and minima, with the most recent glacial maximum occurring around 20,000 years ago. The subsequent warming led to the current interglacial period, characterized by relatively stable and warm conditions. Understanding these historical patterns is essential for predicting future climate trends. By analyzing past ice core data, sediment cores, and other geological records, scientists can reconstruct past climates and identify recurring patterns. For instance, the 40,000-year and 100,000-year cycles in Earth's orbital parameters are closely correlated with glacial cycles. However, human activities, particularly the emission of greenhouse gases, have significantly altered the natural climate cycle, making it challenging to predict when the next ice age might occur. Despite these complexities, studying historical ice age patterns provides valuable insights into Earth's climate system and its natural variability. This knowledge helps scientists to better understand how human-induced climate change interacts with natural climate cycles, ultimately informing strategies for mitigating and adapting to future climate changes. As we continue to monitor Earth's climate and refine our understanding of its dynamics, we move closer to answering the question of when the next ice age might happen, or if human activities have altered this cycle indefinitely.
Role of Orbital Variations
Orbital variations play a pivotal role in understanding the ice age cycle, as they significantly influence Earth's climate by altering the distribution and intensity of solar energy received by our planet. These variations are primarily driven by changes in Earth's orbital parameters, including its eccentricity (the shape of its orbit around the Sun), obliquity (the tilt of its axis), and precession (the wobble of its axis). **Eccentricity** affects the total amount of solar energy Earth receives over the course of a year. When Earth's orbit is more elliptical, the difference between the amount of solar energy received at perihelion (closest point to the Sun) and aphelion (farthest point) is greater, which can lead to more pronounced seasonal variations. This, in turn, can impact global temperatures and ice sheet formation. **Obliquity**, or axial tilt, influences the distribution of solar energy between the equator and the poles. A higher tilt results in more extreme seasons, with colder winters and warmer summers. This increased seasonal contrast can lead to the expansion of ice sheets during colder periods. **Precession** affects the timing of perihelion and aphelion relative to Earth's seasons. When perihelion occurs during the Northern Hemisphere's summer, it can lead to warmer summers and cooler winters, potentially melting ice sheets. Conversely, when perihelion occurs during the Northern Hemisphere's winter, it can result in colder summers and warmer winters, favoring ice sheet growth. The interplay of these orbital variations, known as Milankovitch cycles, is crucial for understanding the onset and termination of ice ages. For instance, during periods when Earth's orbit is less eccentric and its axial tilt is lower, less solar energy reaches the Northern Hemisphere during summer months, making it more likely for ice sheets to expand and persist. Conversely, when these conditions are reversed, increased summer insolation can lead to ice sheet melting and interglacial periods. In summary, orbital variations are key drivers of climate change on geological timescales, influencing the formation and melting of ice sheets through their impact on solar energy distribution. Understanding these cycles is essential for predicting future climate trends and determining when the next ice age might occur. By analyzing past patterns and current orbital configurations, scientists can better forecast Earth's future climate trajectory and the potential timing of future glacial cycles.
Impact of Volcanic Eruptions
Volcanic eruptions play a significant role in the Earth's climate system, particularly in the context of understanding the Ice Age cycle. These powerful events can have profound impacts on global temperatures and atmospheric conditions, influencing the onset and duration of ice ages. When a volcano erupts, it releases massive amounts of ash, sulfur dioxide, and other gases into the atmosphere. These particles can reflect sunlight back into space, reducing the amount of solar radiation that reaches the Earth's surface. This phenomenon, known as a "volcanic winter," can lead to a temporary cooling of the planet. Over extended periods, repeated volcanic eruptions can contribute to a sustained cooling effect, potentially triggering or exacerbating an ice age. For instance, during the Little Ice Age (1550-1850 AD), a series of large volcanic eruptions is believed to have contributed to the cooler global temperatures observed during that time. The sulfur dioxide emitted by these eruptions forms sulfate aerosols in the stratosphere, which remain for several years and continue to reflect sunlight away from Earth. Moreover, volcanic activity can also affect ocean currents and atmospheric circulation patterns. Changes in these systems can alter regional climate conditions, sometimes leading to cooler temperatures in certain areas while warming others. This complex interplay between volcanic activity and climate dynamics underscores the importance of considering these eruptions when predicting future climate trends, including the potential onset of another ice age. In addition to their direct cooling effects, volcanic eruptions can influence long-term climate variability by altering the Earth's energy balance. For example, large eruptions can impact the formation of ice sheets by creating conditions that favor ice accumulation. This feedback loop between volcanic activity and ice sheet formation highlights the intricate relationships within Earth's climate system and emphasizes the need for comprehensive models that account for these interactions when forecasting future climatic changes. Understanding the impact of volcanic eruptions on climate is crucial for predicting when the next ice age might occur. While human activities such as greenhouse gas emissions currently dominate discussions about climate change, natural factors like volcanic eruptions remain significant contributors to Earth's climatic evolution. By integrating data on past volcanic events with modern climate modeling techniques, scientists can better anticipate how these natural disturbances might influence future climatic shifts, including those that could lead to another ice age. This holistic approach ensures a more accurate understanding of Earth's complex climate system and its potential future trajectories.
Current Climate Trends and Predictions
In the face of escalating environmental concerns, understanding current climate trends and predictions has become paramount. The Earth's climate is undergoing significant changes, driven by various factors that necessitate a comprehensive analysis. This article delves into three critical aspects: **Global Warming and Its Effects**, which explores the immediate and long-term impacts of rising temperatures on ecosystems and human societies; **Climate Models and Projections**, which examines the sophisticated tools used to forecast future climate scenarios; and **Observations from Paleoclimatology**, which draws insights from ancient climates to contextualize current trends. By examining these interconnected elements, we gain a deeper understanding of the complex dynamics shaping our planet's climate. This knowledge is crucial not only for mitigating the adverse effects of climate change but also for appreciating broader climatic cycles, such as those that govern the onset and retreat of ice ages. As we navigate these pressing issues, it becomes clear that grasping current climate trends is essential for **Understanding the Ice Age Cycle**, a topic that will be explored in greater detail following this examination of contemporary climate dynamics.
Global Warming and Its Effects
Global warming, a pressing issue of our time, is characterized by the steady increase in Earth's average surface temperature due to human activities that release greenhouse gases, such as carbon dioxide and methane, into the atmosphere. This phenomenon has profound effects on various aspects of our planet and its ecosystems. One of the most immediate consequences is the rise in sea levels, primarily caused by the melting of polar ice caps and glaciers. This not only threatens coastal communities with increased flooding and erosion but also exacerbates saltwater intrusion into freshwater sources, compromising drinking water supplies and agricultural productivity. Climate change also alters weather patterns, leading to more frequent and intense natural disasters like hurricanes, droughts, and wildfires. These extreme events disrupt ecosystems, causing widespread loss of biodiversity as species struggle to adapt to rapidly changing environmental conditions. For instance, shifts in temperature and precipitation patterns can decimate crops, impacting global food security and economic stability. Additionally, warmer temperatures facilitate the spread of diseases and pests, posing significant health risks to both humans and wildlife. The impact on human health is multifaceted; warmer temperatures increase heat-related illnesses, while changes in weather patterns can lead to increased pollen production, exacerbating respiratory issues like asthma. Furthermore, climate change influences the distribution of disease vectors such as mosquitoes, which can spread diseases like malaria and dengue fever to new regions. Economically, global warming imposes substantial costs through damage to infrastructure, loss of property, and impacts on tourism and recreation industries. The melting of permafrost in Arctic regions releases methane, a potent greenhouse gas, creating a self-reinforcing cycle that accelerates warming. This feedback loop underscores the urgent need for global cooperation to reduce emissions and transition to renewable energy sources. In the context of current climate trends and predictions, understanding the effects of global warming is crucial for developing strategies to mitigate its impacts. While some argue that natural climate variability could eventually lead to another ice age, scientific consensus overwhelmingly supports the notion that human-induced warming will dominate climate trends for centuries to come. Therefore, addressing global warming through sustainable practices and policy changes is essential for ensuring a livable future for generations to come.