When The Rain Stops
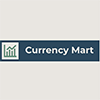
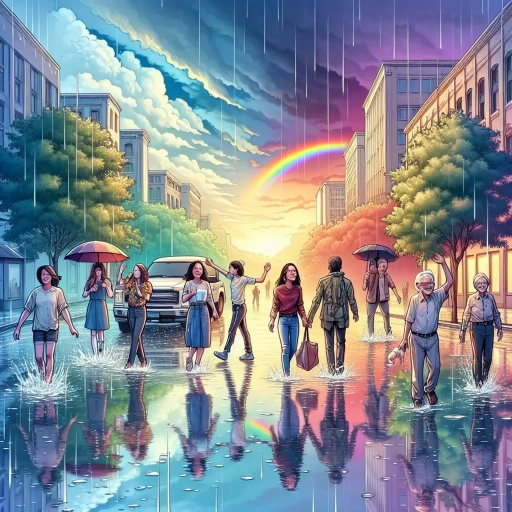
The cessation of rainfall is a moment of relief and renewal, marking the end of a period that can be both nourishing and disruptive. Understanding when the rain stops is crucial for various aspects of life, from agriculture and urban planning to daily routines and outdoor activities. This article delves into the intricacies of rainfall cessation, exploring three key areas: **Understanding Rain Cycles and Patterns**, which examines the natural cycles that govern precipitation; **Factors Influencing the End of Rainfall**, which discusses the environmental and climatic elements that determine when rain stops; and **Predicting When Rain Will Stop**, which looks at the scientific methods used to forecast the end of rainfall. By grasping these concepts, we can better prepare for and respond to changes in weather patterns. Let's begin by exploring the fundamental **Understanding Rain Cycles and Patterns**, which forms the basis of our comprehension of rainfall dynamics.
Understanding Rain Cycles and Patterns
Understanding rain cycles and patterns is crucial for grasping the intricate dynamics of Earth's hydrological system. Rainfall, a vital component of the water cycle, varies significantly across different regions and seasons, influencing ecosystems, agriculture, and human settlements. This article delves into the complexities of rain cycles by examining three key aspects: **Global Rainfall Distribution**, which highlights the uneven distribution of rainfall around the globe and its implications for regional climates; **Seasonal Variations in Rainfall**, which explores how rainfall patterns change throughout the year due to shifts in atmospheric conditions; and **Impact of Climate Change on Rainfall**, which discusses how global warming is altering traditional rainfall patterns, leading to more frequent extremes. By understanding these facets, we can better appreciate the mechanisms behind rain cycles and their critical role in sustaining life on Earth. This comprehensive overview will provide insights into the multifaceted nature of rainfall, ultimately enhancing our understanding of rain cycles and patterns.
Global Rainfall Distribution
Global rainfall distribution is a complex and dynamic phenomenon that plays a crucial role in shaping Earth's climate, ecosystems, and human societies. The distribution of rainfall varies significantly across different regions due to several key factors, including latitude, altitude, ocean currents, and the presence of mountain ranges. Near the equator, the Intertropical Convergence Zone (ITCZ) is characterized by high levels of rainfall due to the convergence of warm, moist air masses from the northern and southern hemispheres. This zone is home to some of the world's most prolific rainforests, such as the Amazon and Congo Basins. In contrast, arid and semi-arid regions like the Sahara Desert and parts of Australia experience very low rainfall due to their location under high-pressure belts where descending air masses suppress cloud formation. Mountain ranges also significantly influence rainfall patterns; for instance, the Himalayas force warm, moist air from the Indian Ocean to rise, cool, and condense, resulting in heavy precipitation on the windward side and creating rain shadows on the leeward side. This orographic effect is evident in regions like the Western Ghats of India and the Andes in South America. Seasonal variations further complicate global rainfall distribution. Monsoon systems, such as those in India and Southeast Asia, bring substantial rainfall during specific months of the year due to changes in wind patterns and temperature gradients. Similarly, tropical cyclones and hurricanes can deliver intense rainfall to coastal areas during their active seasons. Understanding these patterns is crucial for predicting weather events, managing water resources, and mitigating the impacts of droughts and floods. Moreover, global rainfall distribution is not static; it is influenced by climate change and other anthropogenic factors. Rising global temperatures alter atmospheric circulation patterns and moisture content, leading to shifts in precipitation zones and intensities. This can result in more frequent extreme weather events, such as heavy downpours and prolonged droughts, which have significant implications for agriculture, urban planning, and environmental conservation. In summary, global rainfall distribution is a multifaceted topic that involves intricate interactions between atmospheric, oceanic, and terrestrial systems. Recognizing these patterns and their underlying drivers is essential for developing effective strategies to manage water resources sustainably and adapt to the challenges posed by climate variability and change. By understanding these cycles and patterns, we can better prepare for the future and ensure resilient ecosystems and communities.
Seasonal Variations in Rainfall
Seasonal variations in rainfall are a critical component of understanding rain cycles and patterns, as they significantly influence the hydrological balance and ecological dynamics of various regions. These variations are primarily driven by changes in atmospheric circulation, temperature, and the position of the Earth relative to the Sun. In tropical regions, such as those near the equator, rainfall often follows a bimodal pattern due to the movement of the Intertropical Convergence Zone (ITCZ), which shifts northward and southward with the seasons. This results in distinct wet and dry seasons, with the wet seasons typically occurring when the ITCZ is overhead. In contrast, mid-latitude regions experience rainfall patterns that are more closely tied to the annual cycle of temperature and atmospheric pressure systems. For instance, areas influenced by the Asian monsoon exhibit pronounced seasonal rainfall due to the land-sea temperature contrast between the Asian continent and the Indian Ocean. During summer, the land heats up faster than the sea, creating a low-pressure system over land that pulls in moist air from the ocean, leading to heavy rainfall. Conversely, during winter, the land cools more rapidly than the sea, reversing the pressure gradient and resulting in dry conditions. In temperate zones, seasonal rainfall is often associated with the movement of high and low-pressure systems. For example, the western coasts of North America and Europe receive significant rainfall during the winter months due to the prevailing westerly winds that bring moist air from the oceans. In these regions, the summer months are generally drier as high-pressure systems dominate, leading to clearer skies and reduced precipitation. Understanding these seasonal variations is crucial for managing water resources, predicting weather patterns, and mitigating the impacts of extreme weather events such as floods and droughts. Farmers rely on these patterns to plan planting and harvesting cycles, while urban planners use this information to design infrastructure that can handle varying levels of rainfall. Additionally, recognizing the seasonal shifts in rainfall helps in monitoring climate change effects, as changes in these patterns can indicate broader climatic trends. Overall, the study of seasonal variations in rainfall provides valuable insights into the complex interactions between atmospheric, terrestrial, and oceanic systems. By analyzing these patterns, scientists can better predict future rainfall trends, which is essential for sustainable development and environmental stewardship. As we continue to navigate the challenges posed by climate variability, understanding the intricacies of seasonal rainfall will remain a cornerstone of hydrological research and practical applications.
Impact of Climate Change on Rainfall
The impact of climate change on rainfall is a complex and multifaceted issue that significantly affects global rain cycles and patterns. As Earth's temperature rises due to increasing greenhouse gas emissions, it alters the hydrological cycle, leading to changes in precipitation distribution and intensity. One of the most notable effects is the exacerbation of extreme weather events. Warmer air can hold more moisture, resulting in heavier rainfall episodes that can lead to severe flooding. Conversely, this same warming trend can also prolong droughts by increasing evaporation rates and altering atmospheric circulation patterns, which in turn disrupts the normal flow of rain-bearing systems. For instance, regions like the Amazon rainforest are experiencing more frequent and intense droughts, while areas such as Southeast Asia are seeing increased instances of heavy monsoon rains. Climate change also influences the timing and duration of rainy seasons. In some regions, the onset of the rainy season is delayed, while in others it arrives earlier than usual. This shift can have devastating consequences for agriculture, as crops are often adapted to specific rainfall schedules. Additionally, changes in precipitation patterns can disrupt ecosystems, affecting the habitats and survival of various plant and animal species that rely on predictable rain cycles. Furthermore, climate change impacts global atmospheric circulation patterns such as El Niño and La Niña events, which play crucial roles in determining rainfall distribution around the world. These events are becoming more frequent and intense under a warming climate, leading to broader impacts on global weather patterns. For example, during an El Niño event, parts of South America experience heavy rainfall while Australia and Southeast Asia face severe droughts. Understanding these changes is essential for developing effective strategies to mitigate and adapt to the impacts of climate change on rainfall. This includes improving weather forecasting systems to better predict extreme weather events, implementing sustainable agricultural practices that are resilient to changing rain patterns, and enhancing water management systems to handle both floods and droughts. By recognizing the intricate relationship between climate change and rainfall, we can take proactive steps to protect vulnerable communities and ecosystems from the adverse effects of these shifts in rain cycles and patterns. Ultimately, addressing these challenges requires a coordinated global effort to reduce greenhouse gas emissions and adapt to the new climate reality.
Factors Influencing the End of Rainfall
The cessation of rainfall is a complex phenomenon influenced by a multitude of factors, each playing a crucial role in the dynamics of weather patterns. Understanding these factors is essential for grasping the intricacies of rain cycles and their impact on our environment. This article delves into three key areas that significantly affect the end of rainfall: atmospheric conditions and pressure systems, topographical effects, and the role of weather fronts. Atmospheric conditions, including changes in temperature and humidity, interact with pressure systems to create or dissipate rain-bearing clouds. Topographical features, such as mountains and valleys, can either enhance or block rainfall through orographic effects. Additionally, weather fronts—boundaries between different air masses—often mark the beginning and end of precipitation events. By examining these elements, we can gain a deeper understanding of how and why rainfall ceases, ultimately shedding light on the broader context of rain cycles and patterns. This knowledge is vital for predicting weather events, managing water resources, and mitigating the impacts of climate change. Transitioning from these specific influences to a broader understanding of rain cycles and patterns will provide a comprehensive view of the hydrological cycle and its significance in our ecosystem.
Atmospheric Conditions and Pressure Systems
Atmospheric conditions and pressure systems play a crucial role in determining the onset and cessation of rainfall. The interplay between these factors is complex, yet understanding their dynamics is essential for predicting when rain will stop. High and low-pressure systems are key drivers of weather patterns. High-pressure systems, characterized by sinking air and clear skies, typically bring stable weather conditions that inhibit the formation of clouds and precipitation. Conversely, low-pressure systems, marked by rising air and cloud formation, are conducive to rainfall as they create areas where moisture can condense into clouds. The movement and interaction of these pressure systems influence the trajectory and duration of rain-bearing weather fronts. For instance, when a low-pressure system moves into an area, it can bring rain due to the upward motion of air that cools and condenses, forming clouds. However, as this system moves out or weakens, the rain will typically cease. Additionally, the presence of fronts—such as cold fronts or warm fronts—can significantly impact rainfall patterns. Cold fronts, which bring cooler air masses, often lead to precipitation as the warm air is forced upwards, cooling and condensing. Warm fronts, on the other hand, tend to produce more gradual and prolonged periods of rain as the warm air rides over the cooler air. Another critical factor is the atmospheric stability and humidity levels. In stable atmospheric conditions, there is little vertical movement of air, which reduces the likelihood of cloud formation and subsequent rainfall. Conversely, unstable conditions with high humidity allow for significant vertical development of clouds, leading to more intense and prolonged rainfall events. Wind patterns also play a role; for example, wind shear can disrupt the organization of rain-bearing systems, leading to their dissipation. Furthermore, the role of atmospheric moisture content cannot be overstated. High levels of atmospheric moisture are necessary for significant rainfall events. As moisture-laden air moves into an area, it sets the stage for precipitation. However, once this moisture is depleted or moves out of the region, the rain will stop. The balance between evaporation from surfaces and condensation in the atmosphere also influences how long rain persists. In summary, the end of rainfall is influenced by a combination of atmospheric conditions and pressure systems. The movement and interaction of high and low-pressure systems, fronts, atmospheric stability, humidity levels, wind patterns, and moisture content all contribute to determining when rain will cease. Understanding these factors is crucial for accurate weather forecasting and predicting the cessation of rainfall events.
Topographical Effects on Rainfall
Topographical effects play a crucial role in shaping rainfall patterns, significantly influencing where and how much rain falls. The interaction between terrain and atmospheric conditions creates diverse microclimates, leading to varied precipitation levels across different regions. Mountains, for instance, act as barriers that force warm, moist air to rise, cool, and condense, resulting in orographic rainfall. This phenomenon is evident in mountainous areas like the Himalayas and the Andes, where heavy precipitation occurs on the windward side due to the forced ascent of air. Conversely, the leeward side often experiences a rain shadow effect, characterized by arid conditions due to the descent of dry air. Valleys and basins also exhibit unique topographical influences on rainfall. These low-lying areas can trap cold air and moisture, leading to fog and mist formation, which may eventually contribute to light drizzle or steady rain. Additionally, coastal regions are affected by sea surface temperatures and the proximity to large bodies of water, which can enhance evaporation and lead to increased rainfall. Islands and coastal mountains often experience high levels of precipitation due to the combination of orographic and maritime influences. In flat plains and plateaus, topographical effects are less pronounced but still significant. Here, rainfall is more evenly distributed and often influenced by broader climatic patterns such as frontal systems and low-pressure areas. However, even in these regions, subtle variations in elevation and land use can affect local rainfall patterns. For example, urban heat islands can alter precipitation by creating microclimates that attract thunderstorms. Understanding these topographical effects is crucial for predicting when and where rainfall will occur, which in turn helps in managing water resources, agriculture, and urban planning. The interplay between terrain and atmospheric conditions not only determines the distribution of rainfall but also impacts the duration and intensity of precipitation events. As such, topography is a key factor influencing the end of rainfall, as it dictates how long and how heavily rain falls in any given area. By recognizing these influences, meteorologists can provide more accurate forecasts and help communities prepare for and respond to varying rainfall conditions.
Weather Fronts and Their Role in Rainfall Cessation
Weather fronts play a crucial role in the cessation of rainfall, serving as pivotal boundaries between different air masses that influence precipitation patterns. When a cold front moves into an area, it brings cooler, denser air that displaces the warmer, less dense air, leading to the formation of clouds and precipitation. However, as the cold front advances, it eventually pushes the warm air upwards and out of the region, causing the rain-bearing clouds to dissipate. Conversely, a warm front, which is characterized by warmer air riding over cooler air, often results in prolonged periods of rain due to the stable layering of air masses. Yet, once the warm front has fully passed through an area, the rain typically ceases as the atmosphere stabilizes under the warmer conditions. The interaction between these fronts and other atmospheric factors can significantly impact when and how rainfall ends. For instance, if a cold front meets a stationary front or a warm front, it can create a complex weather system known as an occluded front. This occlusion can lead to a prolonged period of rain as the fronts interact and the air masses mix, but ultimately, the rain will cease once the occlusion has moved through the area and the air masses have stabilized. Additionally, the strength and speed of these fronts can influence the duration and intensity of rainfall. A fast-moving cold front might bring heavy rain followed by rapid clearing, while a slow-moving warm front could result in light to moderate rain over an extended period. Topography also plays a role; mountain ranges can force air to rise, cool, and condense, leading to orographic rainfall that may persist until the front has fully traversed the region. In summary, weather fronts are key determinants in the cessation of rainfall. The type of front, its speed and strength, and its interaction with other atmospheric conditions all contribute to when and how rain stops. Understanding these dynamics is essential for predicting weather patterns and anticipating the end of rainfall events. By analyzing the movement and interaction of weather fronts, meteorologists can provide more accurate forecasts, helping communities prepare for and respond to changing weather conditions. This knowledge not only aids in daily planning but also in managing water resources and mitigating the impacts of extreme weather events.
Predicting When Rain Will Stop
Predicting when rain will stop is a complex task that has captivated meteorologists and the general public alike for centuries. With the advent of advanced technologies and refined scientific methods, we now have multiple avenues to forecast the cessation of rainfall. This article delves into three key approaches that enhance our ability to predict when the skies will clear. First, we explore the role of **Meteorological Models and Forecasts**, which leverage sophisticated algorithms and historical data to provide accurate predictions. Next, we examine **Observing Local Weather Indicators**, highlighting how traditional observations of cloud patterns, wind direction, and other environmental cues can offer valuable insights. Finally, we discuss **Technological Tools for Rainfall Prediction**, including radar systems, satellite imagery, and mobile apps that utilize real-time data to forecast rain cessation. By understanding these methods, we can better grasp the intricacies of rain cycles and patterns, ultimately enhancing our ability to predict when the rain will stop. This knowledge not only aids in daily planning but also contributes significantly to **Understanding Rain Cycles and Patterns**.
Using Meteorological Models and Forecasts
When predicting when rain will stop, meteorological models and forecasts play a crucial role in providing accurate and reliable information. These models utilize complex algorithms and vast datasets to simulate atmospheric conditions, allowing meteorologists to forecast weather patterns with increasing precision. The primary tools in this arsenal include numerical weather prediction (NWP) models such as the Global Forecast System (GFS) and the European Centre for Medium-Range Weather Forecasts (ECMWF) model, which are renowned for their accuracy in predicting short-term to medium-term weather phenomena. These models integrate data from a variety of sources, including satellite imagery, radar systems, weather stations, and upper-air observations. By analyzing this data, meteorologists can identify key factors influencing the weather system, such as high and low-pressure systems, fronts, and moisture content. For instance, if a low-pressure system is expected to move out of an area, the model can predict the timing and trajectory of this movement, thereby indicating when the associated rainfall is likely to cease. In addition to NWP models, ensemble forecasting techniques are also employed to account for uncertainties in weather prediction. Ensemble models run multiple simulations with slightly different initial conditions to generate a range of possible outcomes. This approach helps in quantifying the probability of different scenarios, allowing for more informed decision-making. For example, if an ensemble forecast suggests a high probability of the rain stopping within a specific time frame, it enhances the confidence in the prediction. Moreover, advancements in high-resolution models and nowcasting techniques have significantly improved the accuracy of short-term forecasts. Nowcasting involves using current weather conditions and recent trends to predict the immediate future weather. This is particularly useful for predicting the cessation of rain over a localized area within a few hours. The integration of these advanced meteorological tools with real-time data from weather radar and satellite imagery enables meteorologists to issue timely and accurate forecasts. For instance, Doppler radar can track the movement and intensity of precipitation in real-time, providing critical information on when and where the rain is likely to stop. Similarly, satellite imagery can help identify larger-scale weather patterns that influence local conditions. In summary, the use of meteorological models and forecasts is essential for predicting when rain will stop. By leveraging NWP models, ensemble forecasting, high-resolution models, and nowcasting techniques, along with real-time data from various sources, meteorologists can provide precise and reliable predictions. These tools not only aid in understanding complex weather systems but also facilitate better planning and decision-making for both everyday activities and critical operations such as emergency management and agriculture. As technology continues to evolve, the accuracy and reliability of these predictions are expected to improve further, enhancing our ability to anticipate and prepare for changes in the weather.
Observing Local Weather Indicators
Observing local weather indicators is a crucial step in predicting when rain will stop, as these signs often provide immediate and reliable clues about impending changes in weather patterns. By paying attention to various natural and environmental cues, individuals can make informed decisions about their daily activities and preparations. One of the most obvious indicators is the movement and behavior of clouds. Dark, thick clouds that are low-hanging and uniform typically signal ongoing rain, while lighter, more dispersed clouds may indicate that the rain is beginning to clear. Additionally, changes in wind direction and speed can be telling; a shift in wind direction often precedes a change in weather, and an increase in wind speed can sometimes signal the end of a rain event. Another significant indicator is the temperature. A drop in temperature can sometimes precede the end of rain, especially if it is accompanied by a decrease in humidity. Observing animal behavior is also insightful; birds flying low to the ground or seeking shelter may indicate that the rain is not yet over, while birds singing or flying higher in the sky could suggest that the rain is nearing its end. Plant life also offers clues; if leaves and flowers are still drooping from rain but not being further saturated, it might indicate that the rain is slowing down. Furthermore, observing the smell of the air can be surprisingly informative. The scent of wet earth and ozone, often referred to as "petrichor," is commonly associated with the onset of rain but can also persist after the rain has stopped, indicating that the atmosphere is still moist but drying out. Listening to sounds such as the intensity of raindrops on surfaces or the presence of thunder can also help predict when the rain will stop; a decrease in the intensity of raindrops or the absence of thunder suggests that the storm is weakening. In addition to these natural indicators, modern tools like weather apps and radar imagery provide real-time data that can complement local observations. However, relying solely on technology can overlook subtle changes that are observable in one's immediate environment. Combining both traditional observation skills with modern technology enhances the accuracy of weather predictions and allows individuals to make more informed decisions about their daily plans when rain is involved. By integrating these various local weather indicators into your observation routine, you can develop a keen sense of when rain is likely to stop, enabling you to plan activities more effectively and stay prepared for any changes in the weather. This holistic approach not only leverages ancient wisdom but also incorporates contemporary tools, making it a robust method for predicting when rain will cease.
Technological Tools for Rainfall Prediction
Predicting when rain will stop is a complex task that has become significantly more accurate with the advent of advanced technological tools. One of the most critical components in rainfall prediction is the use of satellite imagery. Geostationary and polar-orbiting satellites equipped with sophisticated sensors capture high-resolution images of cloud formations, precipitation patterns, and atmospheric conditions. These images are then analyzed using advanced algorithms to predict the trajectory and intensity of rainfall systems. For instance, satellites like GOES (Geostationary Operational Environmental Satellite) and MODIS (Moderate Resolution Imaging Spectroradiometer) provide real-time data that help meteorologists track storms and predict their future behavior. Another key tool is radar technology, which uses radio waves to detect precipitation and other weather phenomena. Doppler radar systems, in particular, can measure the velocity of particles within storms, allowing forecasters to identify areas of heavy rain, hail, and even tornadoes. This information is crucial for issuing timely warnings and predicting the duration of rainfall events. Additionally, radar data can be combined with satellite imagery to create detailed three-dimensional models of weather systems, enhancing the accuracy of predictions. Ground-based weather stations also play a vital role in rainfall prediction. These stations collect data on temperature, humidity, wind speed, and other atmospheric conditions that are essential for understanding local weather patterns. This data is often integrated into larger weather forecasting models to improve their accuracy. Furthermore, the proliferation of personal weather stations and crowd-sourced weather data through mobile apps has expanded the network of ground-based observations, providing more granular and localized weather information. Computational models are another cornerstone of modern rainfall prediction. Numerical weather prediction (NWP) models, such as the Global Forecast System (GFS) and the European Centre for Medium-Range Weather Forecasts (ECMWF) model, use complex algorithms and vast amounts of data to simulate future weather conditions. These models can forecast rainfall patterns over various time scales, from a few hours to several days, by solving equations that describe atmospheric dynamics. The continuous improvement in computational power and the integration of machine learning techniques have significantly enhanced the accuracy of these models. Lastly, the integration of artificial intelligence (AI) and machine learning (ML) into weather forecasting has revolutionized the field. AI algorithms can analyze vast datasets from multiple sources—satellites, radar, weather stations—to identify patterns that may not be apparent through traditional methods. This allows for more precise predictions and better understanding of complex weather phenomena. For example, ML models can predict the likelihood of heavy rainfall or flash flooding based on historical data and real-time observations. In summary, the combination of satellite imagery, radar technology, ground-based weather stations, computational models, and AI/ML techniques has transformed the landscape of rainfall prediction. These technological tools enable meteorologists to provide more accurate and timely forecasts, helping communities prepare for and respond to rainfall events effectively. As these technologies continue to evolve, the ability to predict when rain will stop will become even more precise, saving lives and reducing economic impacts associated with severe weather events.