Where Does Metal Come From
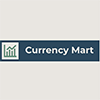
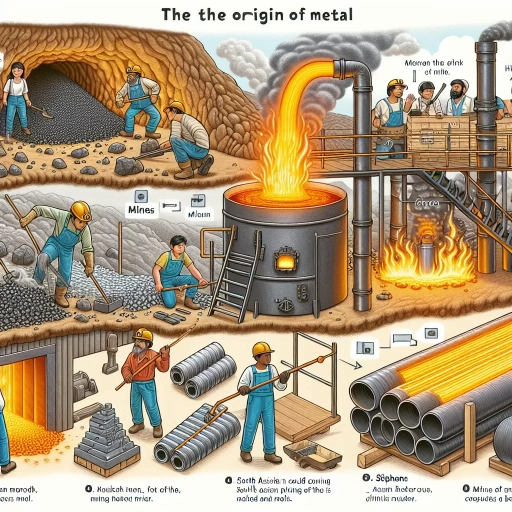
Metal, a fundamental material in modern society, has been a cornerstone of human progress for millennia. From the tools of ancient civilizations to the sophisticated technologies of today, metals have played an indispensable role in shaping our world. But where do these essential resources come from? The journey of metal from its natural state to its final form involves several critical stages. First, understanding the geological origins of metals is crucial; this knowledge helps us identify where and how these elements are naturally formed within the Earth's crust. Next, extraction and mining techniques are employed to retrieve these metals from their natural deposits. Finally, processing and refining metals transforms raw materials into usable products that meet specific industrial standards. In this article, we will delve into each of these stages, starting with the geological origins of metals to uncover the fascinating story behind one of humanity's most valuable resources.
Geological Origins of Metals
Metals are integral to modern society, underpinning everything from technology and infrastructure to healthcare and consumer goods. However, the origins of these essential resources are often overlooked. The geological origins of metals are a fascinating and complex topic that involves various processes occurring deep within Earth's crust. This article delves into the formation of metals in Earth's crust, exploring how tectonic movements and chemical reactions create mineral deposits. It also examines the crucial role of volcanic activity in concentrating metals through magma and volcanic ash. Additionally, it discusses the deposition processes that lead to the formation of economic ore bodies. By understanding these geological mechanisms, we gain insight into the natural history of metals and their availability for human use. This knowledge is vital for sustainable resource management and future exploration efforts. In this article, we will explore the geological origins of metals in depth, shedding light on their formation, volcanic influences, and deposition processes to provide a comprehensive understanding of these valuable resources.
Formation in Earth's Crust
The formation of Earth's crust is a complex and dynamic process that has shaped the planet over billions of years. It begins with the differentiation of the Earth, where heavier elements like iron and nickel sink to the core, while lighter materials such as silicates rise to form the crust. This initial separation sets the stage for subsequent geological processes that contribute to metal formation. One key mechanism is plate tectonics, where moving plates create zones of subduction and collision that drive magma generation and volcanic activity. These magmatic events concentrate metals in economic deposits through processes like fractional crystallization and hydrothermal activity. For instance, copper and gold are often found in porphyry deposits formed by cooling magma chambers beneath volcanic arcs, while nickel and platinum group metals (PGMs) are concentrated in layered igneous intrusions like those found in South Africa's Bushveld Complex. Weathering and erosion also play crucial roles in metal formation by breaking down rocks into sediments that can be transported away from their source areas. These sediments may accumulate in basins where they undergo diagenesis, transforming into sedimentary rocks that can host significant metal deposits. Iron ore deposits, for example, are frequently found in banded iron formations (BIFs), which are layered sedimentary rocks formed through ancient oceanic chemical precipitation processes. Metamorphism further modifies the Earth's crust by subjecting existing rocks to high pressure and temperature conditions that can lead to mineral recrystallization and new mineral formation. This process is particularly important for metals like tungsten and tin, which are often concentrated in metamorphic veins within mountain-building regions. Lastly, biological activity contributes significantly to metal formation through biogeochemical cycles involving microorganisms that facilitate oxidation-reduction reactions essential for depositing metals such as copper and uranium. In summary, the formation of Earth's crust involves a multifaceted interplay between magmatic activity, tectonic movements, weathering processes, metamorphism, and biological interactions—all contributing to the diverse array of metal deposits we exploit today. Understanding these geological origins provides valuable insights into where metals come from and how they are concentrated in economically viable deposits.
Role of Volcanic Activity
Volcanic activity plays a pivotal role in the geological origins of metals, contributing significantly to their formation and distribution within the Earth's crust. Volcanoes are conduits for magma, which is a molten mixture of minerals, gases, and volatiles that ascend from the Earth's mantle. As magma rises, it cools and solidifies, forming igneous rocks that can be rich in metallic elements such as copper, gold, silver, and nickel. The process of magmatic differentiation allows heavier minerals to settle at the bottom of magma chambers while lighter ones rise to the top, leading to the concentration of metals in specific layers or veins. Hydrothermal veins are another critical outcome of volcanic activity. These veins form when hot water rich in dissolved minerals circulates through fractures in rocks near volcanic regions. As this mineral-laden water cools down or reacts with cooler rocks, it precipitates out valuable metals like copper, zinc, lead, and gold. This process is responsible for many economically significant mineral deposits found around volcanic arcs and hotspots. Additionally, volcanic eruptions can deposit ash and other pyroclastic material that may contain trace amounts of metals. Over time, these deposits can accumulate and become concentrated through weathering processes or further geological activity. For instance, volcanic ash can be transported by rivers and deposited in sedimentary basins where they may eventually form metal-bearing sedimentary rocks. The interaction between magma and groundwater also leads to the formation of economic deposits through epithermal mineralization. In these systems, hot fluids interact with cooler rocks at shallow depths beneath volcanoes or volcanic fields. This interaction results in the precipitation of precious metals like gold and silver along with base metals such as copper and zinc. In summary, volcanic activity is essential for creating environments conducive to metal formation through various geological processes including magmatic differentiation, hydrothermal vein formation, pyroclastic deposition, and epithermal mineralization. These processes not only concentrate metals but also provide pathways for their eventual extraction by humans through mining activities. Understanding these mechanisms is crucial for identifying potential mineral resources and managing their extraction sustainably while minimizing environmental impacts.
Deposition Processes
Deposition processes are crucial in the geological origins of metals, as they determine how and where these valuable resources accumulate. These processes involve the transformation of metal-bearing solutions into solid deposits through various mechanisms such as precipitation, sedimentation, and magmatic differentiation. In hydrothermal veins, hot mineral-rich fluids circulate through rock fractures, depositing metals like copper, gold, and silver as they cool or react with the surrounding rock. Similarly, in sedimentary basins, metals can be concentrated through chemical precipitation or biological activity, forming economic deposits of iron ore or manganese nodules. Magmatic differentiation occurs in igneous rocks where molten magma cools and solidifies; heavier minerals like nickel and platinum settle at the base of magma chambers to form layered intrusions. Additionally, weathering and erosion play significant roles by breaking down existing rocks and transporting metal-rich sediments to new locations where they can accumulate in alluvial deposits or placer deposits. Understanding these deposition processes is essential for identifying potential metal sources and developing effective extraction strategies.
Extraction and Mining Techniques
Extraction and mining techniques are pivotal in the global economy, providing essential resources for various industries. These methods have evolved significantly over time, driven by technological advancements and environmental considerations. Open-pit mining methods offer efficient extraction of minerals from surface deposits, while underground mining practices delve into deeper reserves with specialized equipment. Advanced extraction technologies, such as robotic systems and data analytics, enhance safety and productivity. Understanding these techniques is crucial for appreciating the geological origins of metals, which form the foundation of modern civilization.
Open-Pit Mining Methods
Open-pit mining is a widely employed method for extracting minerals and metals from the earth, particularly for deposits that are close to the surface. This technique involves removing soil, rock, and other materials to expose the underlying ore body. The process begins with exploration and planning, where geologists use various techniques such as drilling and sampling to determine the size and quality of the mineral deposit. Once a deposit is identified as economically viable, engineers design an open-pit mine that maximizes extraction efficiency while minimizing environmental impact. The actual mining process typically starts with stripping away overburden—the soil, rock, and other materials covering the ore—to expose it. This is often done using heavy machinery like draglines or hydraulic excavators. The exposed ore is then broken down into manageable pieces using explosives or mechanical tools before being loaded onto trucks or conveyor belts for transportation to processing facilities. One of the key advantages of open-pit mining is its cost-effectiveness compared to underground mining methods. It allows for large-scale operations with high production rates, making it ideal for extracting large volumes of minerals like iron ore, copper, gold, and coal. Additionally, open-pit mines can be designed to minimize environmental disruption by implementing reclamation plans that restore ecosystems after mining activities cease. However, open-pit mining also has significant environmental implications. The removal of vast amounts of overburden can lead to deforestation and habitat destruction. Moreover, water pollution can occur if chemicals used in processing leach into nearby water sources or if acidic mine drainage forms due to exposure of sulfide minerals. Despite these challenges, advancements in technology have improved both efficiency and sustainability in open-pit mining operations. Modern practices include using precision drilling techniques to reduce waste rock removal and employing renewable energy sources to power machinery. Furthermore, many mines now incorporate rigorous environmental monitoring systems to detect potential issues early on. In conclusion, open-pit mining remains a crucial method in the extraction of metals due to its scalability and economic viability. While it presents several environmental challenges that must be carefully managed through responsible practices and technological innovations, it continues to play a vital role in meeting global demands for essential minerals and metals.
Underground Mining Practices
Underground mining practices are a crucial component of metal extraction, offering a method to access valuable minerals and metals located beneath the Earth's surface. This technique involves excavating tunnels and shafts to reach deposits that are not accessible through surface mining. The process begins with exploration, where geologists use various techniques such as drilling and sampling to identify potential mineral deposits. Once a deposit is confirmed, engineers design the mine layout, taking into account factors like rock stability, ventilation requirements, and safety protocols. The actual mining process can be divided into several stages. Room and pillar mining is one common method where large rooms are excavated while leaving behind pillars of rock to support the roof. Another technique is longwall mining, which involves extracting coal or minerals in long horizontal seams using specialized machinery that moves along the face of the deposit. Sublevel caving is another approach where ore is extracted from beneath an overlying rock layer that collapses into the mined-out area. Safety is paramount in underground mining due to risks such as rockfalls, gas explosions, and toxic fumes. Ventilation systems are essential to remove harmful gases and provide breathable air for miners. Advanced technologies like remote-controlled machinery and automated systems enhance efficiency while reducing human exposure to hazardous conditions. Environmental considerations also play a significant role in modern underground mining practices. Efforts are made to minimize environmental impact through careful planning of waste disposal and rehabilitation of mined areas post-extraction. Additionally, many mines now incorporate sustainable practices such as using renewable energy sources for power generation. In conclusion, underground mining practices represent a sophisticated blend of traditional techniques with cutting-edge technology aimed at maximizing resource extraction while ensuring safety and environmental stewardship. As global demand for metals continues to rise, these practices will remain vital for meeting industrial needs while preserving our planet's natural resources for future generations.
Advanced Extraction Technologies
Advanced extraction technologies have revolutionized the mining industry by enhancing efficiency, reducing environmental impact, and improving safety. One of the most significant advancements is the use of autonomous vehicles and drones. These machines can navigate through mines with precision, collecting data on ore quality and quantity in real-time. This data is then used to optimize extraction processes, ensuring that only high-grade ore is extracted while minimizing waste. Another critical technology is hydraulic fracturing (fracking), which has transformed the extraction of metals from hard-to-reach deposits. By injecting high-pressure fluids into rock formations, fracking allows for the release of trapped minerals that were previously inaccessible. This method has significantly increased metal production without the need for extensive drilling or excavation. Robotic mining systems are also gaining prominence. Equipped with advanced sensors and AI algorithms, these robots can perform tasks such as drilling, blasting, and hauling with greater accuracy and speed than human operators. They operate around the clock without breaks, leading to increased productivity and reduced labor costs. In addition to these physical technologies, advanced software solutions play a crucial role in modern mining operations. Geospatial analysis tools help map mineral deposits more accurately than ever before. These tools use satellite imagery and ground-penetrating radar to identify potential sites for extraction before any physical work begins. Sustainable practices are also being integrated into advanced extraction technologies. For instance, bioleaching uses microorganisms to break down ore into its constituent metals without the need for harsh chemicals or high temperatures. This method not only reduces environmental pollution but also lowers energy consumption. Furthermore, nanotechnology is being explored for its potential in improving metal recovery rates from ores. By creating nanoparticles that can selectively bind to specific metals within an ore body, nanotechnology enhances the efficiency of separation processes during refining stages. Lastly, blockchain technology is being adopted to ensure transparency throughout the supply chain—from extraction to end-use products. By tracking each stage of metal production through blockchain ledgers, companies can verify the origin of their materials and ensure compliance with ethical sourcing standards. In conclusion, advanced extraction technologies are transforming how metals are sourced by making processes more efficient, sustainable, and safe. As these technologies continue to evolve with advancements in robotics, software analytics, biotechnology, nanotechnology, and blockchain integration; they promise a future where metal production meets both economic demands and environmental responsibilities effectively.
Processing and Refining Metals
Processing and refining metals is a complex, multifaceted industry that transforms raw materials into valuable commodities. This article delves into the intricacies of metal processing, exploring three critical aspects: smelting and purification processes, alloying and strengthening techniques, and recycling and reuse strategies. We will examine how smelting and purification methods ensure the extraction of pure metals from ores, discuss the science behind alloying to enhance metal properties, and highlight innovative approaches to recycling metals for sustainable use. Understanding these processes not only underscores their industrial significance but also bridges the gap between geological origins of metals and their practical applications in modern society.
Smelting and Purification Processes
Smelting and purification processes are crucial steps in the journey of metals from raw ore to refined product. Smelting involves heating the ore to high temperatures, typically in a furnace, to extract the metal from its mineral compounds. This process often requires the addition of a reducing agent, such as carbon or hydrogen, to remove oxygen and other impurities. The resulting molten metal is then tapped off and further refined through various purification techniques. One common method for purification is electrolysis, where an electric current is passed through the molten metal to separate it from impurities. Another technique is fractional distillation, which leverages differences in boiling points to isolate different elements within a mixture. For certain metals like aluminum and titanium, electrolytic reduction processes are employed due to their high reactivity with oxygen. In addition to these methods, chemical treatments can also be used for purification. For instance, in the production of high-purity metals like silicon for semiconductors, chemical vapor deposition (CVD) can be utilized. This involves reacting volatile compounds with hydrogen at high temperatures to deposit pure silicon onto a substrate. The choice of purification technique depends on the specific properties of the metal being processed and the desired level of purity required for its intended application. For example, precious metals like gold and silver often undergo fire assay—a process involving heating them with lead—to achieve high levels of purity. Overall, smelting and purification processes are essential for transforming raw ores into usable metals that meet industry standards for quality and performance. These techniques not only enhance the physical properties but also ensure that metals are safe for use in various applications ranging from construction materials to electronic components.
Alloying and Strengthening Techniques
Alloying and strengthening techniques are pivotal in the processing and refining of metals, as they significantly enhance the mechanical properties and performance of metallic materials. Alloying involves combining two or more elements to create a new material with superior characteristics compared to its individual components. For instance, steel is an alloy of iron and carbon, which offers greater strength and durability than pure iron. The addition of other elements such as chromium, nickel, or molybdenum can further tailor the properties of steel for specific applications like corrosion resistance in stainless steel or high-temperature resistance in tool steels. Strengthening techniques complement alloying by introducing structural changes that improve the material's strength without altering its chemical composition. One common method is cold working, where metals are subjected to plastic deformation at room temperature through processes like rolling, drawing, or forging. This process introduces dislocations in the crystal structure that impede slip planes and thus enhance hardness and tensile strength. Another technique is heat treatment, which involves controlled heating and cooling cycles to manipulate the microstructure of metals. For example, quenching followed by tempering can achieve a balance between hardness and toughness in steels by forming martensite—a hard but brittle phase—and then relieving internal stresses through tempering. Precipitation hardening is another effective strengthening method used in alloys like aluminum and titanium. This process involves heating the alloy to form precipitates within its matrix, which act as obstacles to dislocation movement during deformation. Additionally, surface treatments such as nitriding or carburizing can enhance wear resistance by forming hard layers on the surface of metals without affecting their bulk properties. In summary, alloying and strengthening techniques are essential tools in metal processing that allow engineers to tailor metallic materials for diverse applications ranging from construction to aerospace engineering. By combining these methods strategically, it is possible to achieve optimal performance characteristics such as strength, ductility, corrosion resistance, and thermal stability—making metals indispensable in modern technology.
Recycling and Reuse Strategies
Recycling and reuse strategies play a pivotal role in the sustainable management of metals, significantly reducing the environmental impact associated with metal extraction and processing. By reusing existing materials, we conserve natural resources, decrease energy consumption, and lower greenhouse gas emissions. For instance, recycling aluminum requires only about 5% of the energy needed to produce new aluminum from raw bauxite ore. This not only saves energy but also reduces the carbon footprint associated with mining and refining processes. Moreover, recycling helps to mitigate the environmental degradation caused by mining activities such as deforestation, water pollution, and soil erosion. For example, recycling copper reduces the need for open-pit mining which can lead to habitat destruction and water contamination. Additionally, recycling metals like steel and iron helps in preserving iron ore reserves for future generations while minimizing waste disposal issues. Effective recycling programs also involve community engagement and education on proper waste segregation practices. This ensures that recyclable materials are collected efficiently without contamination. Advanced technologies in recycling facilities enable better sorting mechanisms that can handle complex mixtures of materials more effectively than ever before. Innovative reuse strategies further enhance sustainability by extending the life cycle of metal products. For example, repurposing old machinery parts or transforming scrap metal into new consumer goods not only reduces landfill waste but also supports creative industries that thrive on upcycling materials. Governments can incentivize these practices through tax benefits or subsidies for companies investing in recycling infrastructure or innovative product design using recycled metals. Ultimately, integrating robust recycling and reuse strategies into our metal management systems is crucial for achieving a circular economy where resources are utilized optimally with minimal waste generation. By adopting these practices globally, we can ensure a more sustainable future where metal production aligns with environmental stewardship without compromising economic growth or technological advancement.