Big Bang Theory Where To Watch Canada
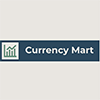
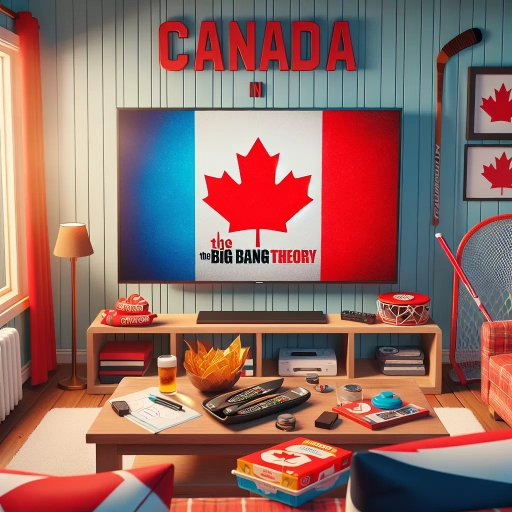
The Big Bang Theory, a cornerstone of modern astrophysics, has captivated scientists and the general public alike with its profound implications about the origin and evolution of our universe. This article delves into three pivotal aspects of the Big Bang Theory: Understanding the Big Bang Theory, Exploring the Timeline of the Universe, and Observational Evidence for the Big Bang. By examining these facets, we gain a comprehensive insight into how our cosmos came to be. First, we will explore what exactly the Big Bang Theory entails—its core principles and how it explains the universe's inception. Next, we will trace the timeline of cosmic events from that initial explosion to present day, highlighting key milestones in universal development. Finally, we will scrutinize observational evidence that supports this theory—data from cosmic microwave background radiation to redshifts in distant galaxies. This journey through time and space begins with Understanding the Big Bang Theory.
Understanding the Big Bang Theory
The Big Bang Theory is the cornerstone of modern cosmology, offering a profound explanation for the origins and evolution of our universe. This theory posits that the universe began as an infinitely hot and dense point approximately 13.8 billion years ago, expanding rapidly into the vast expanse we observe today. To delve into this concept, it is essential to explore its foundational aspects: the origins of the universe, which trace back to a singular event that set everything in motion; key evidence supporting the theory, including cosmic microwave background radiation and redshifted light from distant galaxies; and scientific consensus and debates, which highlight ongoing research and discussions among experts. By examining these elements, we can gain a deeper understanding of how our universe came to be and why the Big Bang Theory remains a pivotal framework in contemporary science. Understanding the Big Bang Theory is crucial for grasping not only where we come from but also where we are headed in our quest for knowledge about the cosmos.
Origins of the Universe
The origins of the universe are deeply intertwined with the Big Bang Theory, which posits that the cosmos began as an infinitely hot and dense point approximately 13.8 billion years ago. This singular event marked the beginning of space and time as we know it, expanding rapidly into the vast expanse we observe today. The Big Bang Theory is supported by a wealth of scientific evidence, including cosmic microwave background radiation, the abundance of light elements, and the large-scale structure of the universe. These phenomena collectively paint a picture of an evolving cosmos that has cooled and expanded over billions of years, giving rise to galaxies, stars, planets, and eventually life itself. Understanding this theory not only provides insights into our cosmic history but also underscores our place within an ever-expanding universe. In Canada, enthusiasts can explore these concepts through various educational programs and documentaries available on platforms like CBC Gem or streaming services such as Netflix and Amazon Prime Video. These resources offer engaging narratives that delve into both theoretical frameworks and observational evidence supporting our current understanding of the universe's origins. By exploring these materials, Canadians can gain a deeper appreciation for how science continues to unravel the mysteries of creation while inspiring new generations to pursue careers in astronomy and cosmology.
Key Evidence Supporting the Theory
The Big Bang Theory, a cornerstone of modern astrophysics, is supported by a plethora of compelling evidence. One key piece of evidence is the Cosmic Microwave Background Radiation (CMB). In the 1960s, scientists Arno Penzias and Robert Wilson discovered a persistent, low-level hum of microwave radiation that permeates the universe. This CMB is thought to be residual heat from the initial explosion, providing strong support for the Big Bang Theory. The uniformity and slight variations in this radiation align perfectly with predictions made by Big Bang models. Another crucial piece of evidence comes from Abundance of Light Elements. According to Big Bang Theory, during the first few minutes after the universe's inception, temperatures were so high that protons and neutrons combined to form light elements such as hydrogen, helium, and lithium. The observed abundance of these elements in the universe matches closely with what would be expected if they were formed in such conditions. The Redshift of Light from Distant Galaxies also plays a significant role in supporting this theory. Astronomers have observed that light coming from distant galaxies is shifted towards the red end of the spectrum—a phenomenon known as redshift. This indicates that these galaxies are moving away from us at high speeds, consistent with an expanding universe—a fundamental aspect of Big Bang cosmology. Additionally, Hubble's Law provides further validation. In 1929, Edwin Hubble observed that galaxies are moving away from each other at speeds proportional to their distance—a direct consequence of an expanding universe originating from a single point. Lastly, Large-scale Structure Formation aligns well with Big Bang predictions. The universe's large-scale structures—such as galaxy clusters and superclusters—can be explained by gravitational collapse within an expanding universe starting from tiny fluctuations present in the CMB. These diverse lines of evidence collectively form a robust framework that substantiates our understanding of how our cosmos began billions of years ago through an immense explosion known as The Big Bang Theory.
Scientific Consensus and Debates
Scientific consensus and debates are integral components of the scientific process, particularly when it comes to understanding complex theories like the Big Bang. The Big Bang Theory, which posits that the universe began as an infinitely hot and dense point approximately 13.8 billion years ago, is widely accepted by the scientific community. This consensus is built on a robust foundation of empirical evidence from various fields such as cosmology, astrophysics, and particle physics. Observations of cosmic microwave background radiation, the abundance of light elements, and the large-scale structure of the universe all support this theory. Despite this broad consensus, scientific debates continue to refine our understanding and address remaining questions. For instance, while the Big Bang explains how the universe expanded and cooled, it does not account for what caused this initial expansion or what existed before it. These open questions fuel ongoing research into theories such as inflationary cosmology and multiverse hypotheses. Moreover, debates within the scientific community often revolve around interpretations of data and theoretical models. For example, some scientists argue over whether dark matter is composed of WIMPs (Weakly Interacting Massive Particles) or axions. These discussions are crucial for advancing our knowledge because they encourage rigorous testing and validation of hypotheses. The dynamic interplay between consensus and debate ensures that scientific theories remain open to revision based on new evidence. This process underscores the self-correcting nature of science: as more data becomes available or new methodologies are developed, existing theories can be refined or even replaced if necessary. In Canada, where there is a strong tradition of scientific research and education, these debates are actively pursued in academic institutions and research centers. Scientists at universities like McGill in Montreal or UBC in Vancouver contribute significantly to our understanding of cosmological phenomena through their work on observational astronomy and theoretical physics. In conclusion, while there is a strong consensus around the Big Bang Theory among scientists globally—including those in Canada—the ongoing debates highlight the dynamic nature of scientific inquiry. These discussions not only deepen our comprehension but also drive innovation in fields related to cosmology. As we continue to explore the mysteries surrounding the origins of our universe through both observation and theoretical work, we move closer to a more complete understanding of how everything began with that singular event known as the Big Bang.
Exploring the Timeline of the Universe
The universe, a vast and intricate tapestry of time and space, has captivated human imagination for centuries. From the earliest moments of its existence to the present day, the universe's timeline is a rich narrative of formation, expansion, and evolution. This article delves into three pivotal stages in this cosmic journey: the early stages where matter and energy first coalesced; the expansion and cooling that characterized the first billion years; and the subsequent formation and evolution of galaxies. By exploring these phases, we gain a deeper understanding of how our universe came to be as we know it today. Ultimately, this exploration leads us to a profound appreciation of the Big Bang Theory, which underpins our modern comprehension of cosmic origins.
Early Stages: Formation of Matter and Energy
In the early stages of the universe, following the Big Bang, matter and energy underwent a series of transformative processes that laid the foundation for the cosmos as we know it today. Approximately 13.8 billion years ago, the universe began as an infinitely hot and dense point known as a singularity. This singularity expanded rapidly in an event known as inflation, smoothing out any irregularities in the universe's fabric. As it expanded, it cooled, allowing for the formation of subatomic particles such as protons, neutrons, and electrons from quarks and leptons. During this period, known as Big Bang nucleosynthesis, protons and neutrons combined to form atomic nuclei—primarily hydrogen and helium—through nuclear reactions. The universe continued to expand and cool further until electrons could combine with these nuclei to form neutral atoms. This era marked a significant milestone: the Cosmic Microwave Background Radiation (CMB), which is still detectable today as residual heat from this early epoch. The next phase saw gravitational forces take hold, causing matter to clump together into larger structures such as galaxies and galaxy clusters. Within these galaxies, gas clouds collapsed under gravity to form stars. These first stars were massive and short-lived; they ended their lives in supernovae explosions that seeded space with heavier elements forged in their cores. These elements are crucial for life on Earth; they include carbon, oxygen, nitrogen, iron—essentials for biological processes—and even heavier metals like gold and uranium produced during supernovae explosions or neutron star mergers. The remnants of these ancient stars also coalesced into new stellar generations along with planetary systems where conditions were ripe for life to emerge. Understanding these early stages is pivotal in Exploring the Timeline of the Universe because they set forth all subsequent cosmic evolution leading up to our present-day universe filled with diverse celestial objects from black holes to exoplanets teeming with potential life forms. By studying this primordial history through observations of distant galaxies or relic radiation like CMBR scientists continue unraveling mysteries about our cosmic origins while inspiring new generations towards deeper exploration into space's vast expanse. For those interested in delving deeper into these topics or witnessing visual representations through documentaries or educational programs there are several platforms available in Canada where one can watch Big Bang Theory related content including streaming services like Netflix which offers documentaries such as "Cosmos" hosted by astrophysicist Neil deGrasse Tyson; educational channels on YouTube featuring lectures by renowned physicists; or even local Canadian television networks airing science-focused programming regularly updated with latest discoveries making it easier than ever before for anyone curious about universe's beginnings explore its wonders right from comfort home
Expansion and Cooling: The First Billion Years
Expansion and Cooling: The First Billion Years In the first billion years following the Big Bang, the universe underwent a transformative period marked by rapid expansion and cooling. This era, often referred to as the "cosmic dawn," was pivotal in shaping the universe as we know it today. Initially, temperatures were so high that matter existed in a state of plasma, with electrons and protons moving freely. As expansion continued, temperatures gradually dropped, allowing for the formation of neutral atoms—a process known as recombination. This transition occurred approximately 380,000 years after the Big Bang and is crucial because it allowed photons to travel freely through space without being scattered by electrons, resulting in what we now observe as the cosmic microwave background radiation (CMB). The CMB serves as a snapshot of this early universe and provides valuable insights into its composition and structure. Following recombination, gravity began to play a more significant role in shaping cosmic structures. Regions with slightly higher densities started to collapse under their own gravitational pull, eventually forming galaxies and galaxy clusters. These early galaxies were likely small and irregular compared to modern spiral or elliptical galaxies but were crucial in seeding subsequent star formation. The first stars and galaxies were primarily composed of hydrogen and helium—the lightest elements formed during Big Bang nucleosynthesis. These stars were massive and short-lived compared to their modern counterparts; they quickly exhausted their fuel through nuclear fusion processes in their cores before ending their lives in spectacular supernovae explosions. These supernovae played a dual role: they dispersed heavy elements synthesized within stellar interiors into interstellar space while also providing kinetic energy that helped shape galaxy morphology. As these heavy elements accumulated over time through successive generations of star formation and supernovae explosions, they paved the way for more complex structures such as planets capable of supporting life. The cooling universe also facilitated molecular formation—hydrogen molecules (H₂) being among the first—setting the stage for further chemical evolution leading up to our current epoch where diverse molecular species are abundant. In summary, during its first billion years, the universe transitioned from an extremely hot plasma state to one where neutral atoms could form; this period laid down foundational elements necessary for later cosmic evolution including galaxy formation and chemical diversification essential for life's emergence on Earth billions of years later.
Galaxy Formation and Evolution
Galaxy formation and evolution are intricate processes that have captivated astronomers for centuries. The journey begins with the Big Bang, approximately 13.8 billion years ago, when the universe was a hot, dense plasma. As it expanded and cooled, matter began to coalesce into the first stars and galaxies. These early galaxies were likely small and irregular, composed of gas and dust that eventually formed stars through gravitational collapse. Over time, these galaxies merged with others in a cosmic dance of collision and accretion, growing larger and more complex. The formation of spiral galaxies like our Milky Way is believed to have occurred through the merger of smaller galaxies. This process led to the creation of central bulges and disk structures where new stars continue to form from interstellar gas. Elliptical galaxies, on the other hand, are thought to result from major mergers that stripped away gas reserves, halting star formation. Galaxy evolution is also influenced by dark matter—a mysterious substance that provides gravitational scaffolding for galaxy structure without emitting light. Dark matter helps hold galaxies together against their own centrifugal forces as they spin. Observational evidence supports these theories: distant galaxies observed by telescopes like Hubble appear younger and more chaotic than those closer to us in space-time. The cosmic microwave background radiation provides further insight into the early universe's conditions conducive to galaxy formation. Understanding galaxy formation and evolution not only sheds light on how our own Milky Way came into existence but also offers a broader perspective on the universe's history and structure. By studying galaxies at various stages of development across vast distances, scientists can reconstruct a timeline of cosmic events that have shaped our universe over billions of years. This knowledge underscores the dynamic nature of galaxies as they evolve through mergers, starbursts, and interactions with their surroundings—each step contributing to their current forms observed today. The study of galaxy formation and evolution remains an active area of research with ongoing missions like NASA's James Webb Space Telescope aiming to uncover even more secrets about these celestial wonders. In summary, galaxy formation and evolution are complex processes driven by gravitational forces acting over immense scales of space and time. From their humble beginnings as small clumps of matter in an expanding universe to their current diverse forms—spiral arms spinning gracefully or elliptical shapes glowing softly—galaxies represent some of nature's most awe-inspiring creations.
Observational Evidence for the Big Bang
The Big Bang Theory is the cornerstone of modern cosmology, providing a comprehensive explanation for the origin and evolution of the universe. Observational evidence plays a crucial role in validating this theory, offering tangible proof that supports its fundamental principles. One key piece of evidence is the Cosmic Microwave Background Radiation, which is the residual heat from the initial explosion. Another significant indicator is the Abundance of Light Elements, such as hydrogen and helium, whose proportions align with predictions made by Big Bang nucleosynthesis. Additionally, the Large-scale Structure of the Universe—galaxies and galaxy clusters—demonstrates a pattern consistent with an expanding universe that began in a singular event. Understanding these observational evidences not only reinforces our confidence in the Big Bang Theory but also deepens our comprehension of how our universe came to be. By examining these phenomena closely, we can gain profound insights into the very fabric of existence and better understand the Big Bang Theory.
Cosmic Microwave Background Radiation
The Cosmic Microwave Background Radiation (CMB) is a pivotal piece of observational evidence supporting the Big Bang Theory. This faint glow of light is the residual heat from the initial explosion that marked the beginning of our universe. Discovered in 1964 by Arno Penzias and Robert Wilson, the CMB is a form of electromagnetic radiation that fills the universe and can be detected in every corner of space. It is a remnant of the early universe, dating back to when it was just 380,000 years old and had cooled enough for electrons and protons to combine into neutral atoms, allowing photons to travel freely through space. The CMB's significance lies in its uniformity and tiny fluctuations. The uniformity suggests that the universe was once extremely hot and dense, consistent with Big Bang predictions. However, tiny variations in temperature across different regions indicate slight differences in density at an early stage, which eventually led to the formation of galaxies and stars. These fluctuations are crucial for understanding how matter clumped together under gravity to form large-scale structures in our cosmos. Observations from satellites like COBE (Cosmic Background Explorer), WMAP (Wilkinson Microwave Anisotropy Probe), and Planck have provided detailed maps of these temperature variations with unprecedented precision. These maps not only confirm predictions made by Big Bang cosmology but also offer insights into fundamental parameters such as the age, composition, and geometry of our universe. Moreover, studies on polarization patterns within the CMB have further reinforced its connection to gravitational waves predicted by inflationary models—a theory suggesting that our universe underwent rapid expansion shortly after its inception. The detection of these polarization patterns by missions like BICEP2 underscores how closely aligned observational data are with theoretical frameworks derived from Big Bang cosmology. In summary, the Cosmic Microwave Background Radiation stands as compelling evidence for the Big Bang Theory. Its discovery has been instrumental in shaping our understanding of cosmic origins while ongoing research continues to refine our knowledge about this ancient light that permeates every corner of existence.
Abundance of Light Elements
The abundance of light elements, such as hydrogen, helium, and lithium, serves as a crucial piece of observational evidence supporting the Big Bang theory. According to the Big Bang model, the universe began as an infinitely hot and dense point approximately 13.8 billion years ago. In the first few minutes after this cosmic inception, known as Big Bang nucleosynthesis (BBN), protons and neutrons combined to form these light elements. The precise ratios of these elements observed in the universe today closely match predictions derived from BBN calculations. For instance, hydrogen is the most abundant element in the universe by mass, followed by helium-4, which makes up about 24% of all baryonic matter. These proportions are consistent with what would have been produced during those initial minutes when temperatures were high enough for nuclear reactions to occur but too low for heavier elements to form. The abundance of light elements also helps rule out alternative theories that suggest a static or cyclic universe. If the universe were static or cyclic, it would likely have undergone numerous cycles of expansion and contraction over billions of years, leading to different elemental compositions due to ongoing stellar processes. However, our observations show a uniform distribution of light elements across vast distances and diverse astrophysical environments—stars, galaxies, interstellar gas clouds—indicating they were formed during a single primordial event rather than through continuous stellar activity. Moreover, the presence of trace amounts of lithium-7 (Li-7) in ancient stars provides further evidence supporting BBN predictions. These stars are thought to have formed early in cosmic history without significant enrichment from later stellar nucleosynthesis processes that could alter their original composition. The observed Li-7 abundance aligns well with theoretical expectations based on Big Bang nucleosynthesis models. In conclusion, the observed abundance of light elements in our universe offers compelling evidence for the Big Bang theory. This phenomenon not only supports our understanding of cosmic origins but also underscores how fundamental physical laws governing nuclear reactions have remained consistent over billions of years. The precise agreement between theoretical predictions and observational data reinforces confidence in our current understanding of how our universe came into being. For those interested in exploring this topic further or learning more about observational evidence for the Big Bang theory in Canada, there are several resources available including documentaries on streaming platforms like Netflix or educational programs on CBC Gem that delve into cosmology and astrophysics topics comprehensively.
Large-scale Structure of the Universe
The large-scale structure of the universe is a fascinating and complex topic that provides significant observational evidence for the Big Bang theory. On a cosmic scale, galaxies are not randomly distributed but are instead organized into vast networks of galaxy clusters, superclusters, and even larger structures known as galaxy filaments. These filaments form a web-like pattern that crisscrosses the universe, with vast voids in between. This intricate structure is a direct result of gravitational forces acting over billions of years, starting from tiny fluctuations in the universe's density during its early stages. Observational evidence supports this hierarchical formation process. The Cosmic Microwave Background Radiation (CMB), which is the residual heat from the Big Bang, shows minute variations in temperature and polarization that correspond to these initial density fluctuations. These variations are thought to have seeded the formation of galaxies and galaxy clusters through gravitational collapse. The Sloan Great Wall, for example, is one such superstructure that stretches over 1.37 billion light-years across space—a testament to how matter has clumped together under gravity's influence. Galaxy surveys like the Sloan Digital Sky Survey (SDSS) have mapped out these large-scale structures with unprecedented detail. By observing how galaxies are distributed and how they move within these structures, scientists can infer much about their evolution and composition. For instance, dark matter plays a crucial role in holding these structures together; its presence can be inferred by observing how galaxies rotate within their clusters and how light bends around massive cosmic objects—a phenomenon known as gravitational lensing. Furthermore, simulations based on Big Bang cosmology accurately predict many features observed in today's universe. These simulations start with conditions similar to those immediately after the Big Bang and evolve them forward in time using known physical laws. They successfully reproduce not only the overall distribution of galaxies but also finer details such as galaxy morphology and star formation rates. In conclusion, the large-scale structure of the universe offers compelling evidence for the Big Bang theory by demonstrating how matter has evolved over billions of years from tiny initial fluctuations into complex networks we see today. This alignment between observation and simulation underscores our understanding that we live in an expanding universe shaped by gravity since its inception around 13.8 billion years ago.