Big Bang Theory Where To Watch
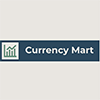
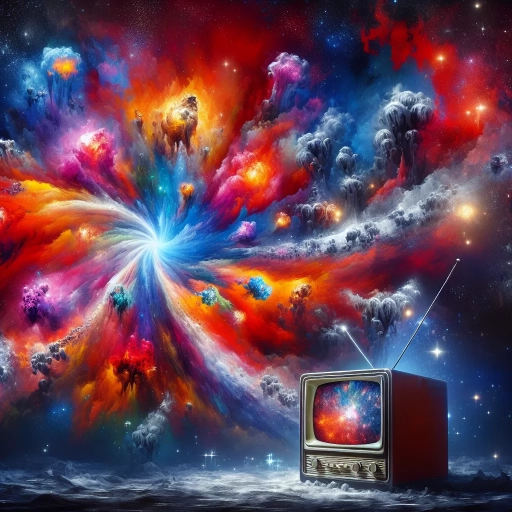
The Big Bang Theory, a cornerstone of modern cosmology, has captivated scientists and the general public alike for decades. This groundbreaking concept posits that the universe began as an infinitely hot and dense point approximately 13.8 billion years ago, expanding rapidly into the vast expanse we observe today. The origins of this theory are deeply rooted in historical scientific discoveries and observations that laid the foundation for our current understanding. Cosmological evidence, including cosmic microwave background radiation and the abundance of light elements, provides robust support for the Big Bang hypothesis. Modern research continues to refine our knowledge, exploring new frontiers in cosmology and shedding light on future directions for study. In this article, we will delve into these aspects starting with The Origins of the Big Bang Theory.
The Origins of the Big Bang Theory
The Big Bang Theory, the leading explanation for the origin and evolution of the universe, has a rich and fascinating history. This article delves into the origins of this groundbreaking theory, exploring early observations and theories that laid its foundation. We will examine the pivotal contributions of key scientists whose work transformed our understanding of the cosmos. Additionally, we will trace the evolution of the Big Bang Theory over time, highlighting significant milestones and advancements that have solidified its status as a cornerstone of modern astrophysics. By understanding these elements—early observations and theories, key scientists and their contributions, and the evolution of the theory over time—we can gain a deeper appreciation for The Origins of the Big Bang Theory.
Early Observations and Theories
The Big Bang Theory, a cornerstone of modern cosmology, has its roots in early observations and theories that laid the groundwork for our current understanding of the universe's origin. In the early 20th century, Belgian priest and cosmologist Georges Lemaitre proposed the "Cosmic Egg" hypothesis, suggesting that the universe began from a single, extremely hot and dense point. This idea was later supported by Edwin Hubble's groundbreaking observations in 1929, which revealed that galaxies are moving away from each other at speeds proportional to their distance—a phenomenon known as Hubble's Law. This expansion led scientists to infer that the universe must have started from a single point and has been expanding ever since. The Big Bang Theory gained further traction with the discovery of cosmic microwave background radiation (CMB) by Arno Penzias and Robert Wilson in 1964. The CMB is thought to be residual heat from the initial explosion, providing strong evidence for the Big Bang model. Additionally, the abundance of light elements such as hydrogen, helium, and lithium can be explained by Big Bang nucleosynthesis—the process by which these elements were formed during the first few minutes after the universe's inception. These early observations and theories collectively formed a robust framework that has been continually refined through subsequent discoveries. For instance, satellite missions like COBE (Cosmic Background Explorer) and WMAP (Wilkinson Microwave Anisotropy Probe) have mapped out minute variations in CMB temperatures across the sky with unprecedented precision. These maps not only confirm predictions made by Big Bang cosmology but also provide insights into fundamental properties of matter and energy. Moreover, advances in particle physics have complemented cosmological findings. The Standard Model of particle physics describes how fundamental particles interacted in conditions similar to those present shortly after the Big Bang. Experiments conducted at high-energy particle colliders aim to recreate these conditions on a microscopic scale, offering insights into what might have occurred during those initial moments. In summary, early observations and theories played a crucial role in shaping our understanding of the Big Bang Theory. From Lemaitre's Cosmic Egg hypothesis to Hubble's Law and Penzias' discovery of CMB radiation, each milestone contributed significantly to our current comprehension of how our universe began. Continued research efforts ensure that this theory remains dynamic and evolving as new data emerges from both observational astronomy and experimental physics.
Key Scientists and Their Contributions
The Big Bang Theory, a cornerstone of modern astrophysics, owes its development to the contributions of several key scientists. One of the earliest and most influential figures is Belgian priest and cosmologist Georges Lemaitre, who in the 1920s proposed the "Cosmic Egg" hypothesis. This idea posited that the universe began from a single, extremely hot and dense point. Lemaitre's work laid foundational groundwork for later theories. Another pivotal figure is Edwin Hubble, an American astronomer who made groundbreaking observations in the 1920s. Hubble discovered that galaxies are moving away from each other at speeds proportional to their distance—a phenomenon known as Hubble's Law. This finding provided empirical evidence for an expanding universe, supporting Lemaitre's initial hypothesis. Theoretical physicist Alan Guth further refined these ideas with his inflationary model of the universe in the early 1980s. Guth proposed that shortly after its inception, the universe underwent a rapid expansion phase known as inflation. This theory helped explain why distant parts of the universe appear so similar despite being separated by vast distances. Additionally, Arno Penzias and Robert Wilson played crucial roles in confirming key aspects of Big Bang Theory through their Nobel Prize-winning discovery of cosmic microwave background radiation (CMB) in 1964. The CMB is residual heat from the initial explosion and serves as strong evidence for the Big Bang. Stephen Hawking also made significant contributions by integrating quantum mechanics into cosmology and proposing black holes emit radiation now known as Hawking radiation. His work on singularities provided deeper insights into how space-time behaves under extreme conditions. Lastly, scientists like Neil deGrasse Tyson have been instrumental in popularizing these concepts through accessible media platforms such as documentaries and podcasts. Their efforts have made complex scientific theories more understandable to a broader audience. In summary, while many scientists have contributed to our understanding of Big Bang Theory over time, these individuals stand out for their transformative insights that have shaped our current understanding of cosmic origins.
Evolution of the Theory Over Time
The evolution of the Big Bang Theory over time has been a dynamic and transformative journey, marked by significant scientific discoveries and theoretical advancements. Initially proposed by Belgian priest and cosmologist Georges Lemaitre in the 1920s, the theory posited that the universe began from a single, extremely hot and dense point. This idea was later supported by Edwin Hubble's observations of galaxy redshifts, which indicated that galaxies are moving away from each other at speeds proportional to their distance—a key piece of evidence for an expanding universe. In the mid-20th century, Arno Penzias and Robert Wilson's accidental discovery of cosmic microwave background radiation (CMB) provided crucial empirical evidence for the Big Bang. The CMB is thought to be residual heat from the early universe, offering a snapshot of conditions just 380,000 years after its inception. This finding solidified the Big Bang as the leading cosmological model. Advancements in technology have continued to refine our understanding. The Cosmic Background Explorer (COBE) satellite launched in 1989 mapped CMB with unprecedented precision, revealing tiny fluctuations that seeded galaxy formation. Subsequent missions like WMAP and Planck further detailed these fluctuations and provided insights into fundamental parameters such as the age and composition of the universe. Theoretical frameworks have also evolved significantly. Inflationary theory, introduced by Alan Guth in 1980, suggests a rapid expansion phase shortly after the Big Bang that explains why distant regions of space appear so similar despite being causally disconnected. This theory has been supported by observations of gravitational waves detected by LIGO and VIRGO collaborations. Moreover, advances in computational power have enabled sophisticated simulations that model cosmic evolution from primordial conditions to present-day structures. These simulations help scientists understand complex processes such as dark matter distribution and galaxy formation dynamics. Today, ongoing research continues to push boundaries with next-generation telescopes like JWST and future missions aimed at detecting primordial gravitational waves—a potential window into even earlier epochs of cosmic history. The Big Bang Theory remains a cornerstone of modern cosmology, its evolution reflecting humanity's relentless pursuit of understanding our origins and place within an ever-expanding universe.
Cosmological Evidence Supporting the Big Bang
The Big Bang Theory, the leading explanation for the origin and evolution of the universe, is supported by a wealth of cosmological evidence. This theory posits that the universe began as an infinitely hot and dense point approximately 13.8 billion years ago and has been expanding ever since. The evidence backing this theory is multifaceted and compelling, encompassing observations such as the Cosmic Microwave Background Radiation, which is the residual heat from the initial explosion; the Abundance of Light Elements, which aligns with predictions from Big Bang nucleosynthesis; and the Large-scale Structure of the Universe, which reflects patterns consistent with early universe conditions. These findings collectively provide strong support for the Big Bang Theory, offering insights into how our cosmos came to be. Understanding these pieces of evidence not only reinforces our current understanding but also sheds light on The Origins of the Big Bang Theory.
Cosmic Microwave Background Radiation
The Cosmic Microwave Background Radiation (CMB) is a pivotal piece of evidence supporting the Big Bang theory. This faint glow of microwave radiation permeates the universe, providing a snapshot of the cosmos when it was just 380,000 years old. The CMB was first discovered by Arno Penzias and Robert Wilson in 1964, who initially thought it was an anomaly caused by pigeon droppings in their antenna. However, further investigation revealed that this uniform radiation was coming from all directions in space, consistent with predictions made by George Gamow and Ralph Alpher about the residual heat from the Big Bang. The CMB's discovery was a groundbreaking moment in cosmology, offering strong empirical support for the Big Bang theory. It shows that the universe began as an infinitely hot and dense point and has been expanding ever since. The radiation itself is thought to be the residual heat from this initial explosion, now cooled to a temperature of about 2.7 degrees Kelvin (-270.42 degrees Celsius). This uniformity across vast distances underscores the universe's homogeneous nature at its inception. Moreover, tiny fluctuations within the CMB provide valuable insights into how galaxies formed over billions of years. These minute variations in temperature and polarization are believed to be seeds for gravitational collapse that eventually led to galaxy formation. The precise mapping of these fluctuations by satellites like COBE (Cosmic Background Explorer), WMAP (Wilkinson Microwave Anisotropy Probe), and Planck have further solidified our understanding of cosmic evolution. In addition to its role in validating the Big Bang theory, the CMB also serves as a tool for understanding fundamental aspects of physics such as dark matter and dark energy—components that make up most of our universe but remain invisible to us directly. By analyzing patterns within the CMB, scientists can infer properties about these mysterious entities that shape our cosmos on large scales. In conclusion, the Cosmic Microwave Background Radiation stands as one of the most compelling pieces of evidence for the Big Bang theory. Its discovery not only confirmed predictions made decades earlier but also opened new avenues for research into cosmic origins and evolution. As scientists continue to refine their understanding through advanced telescopes and missions like Simons Observatory or CMB-S4, they are likely to uncover even more secrets hidden within this ancient light from our universe's earliest moments.
Abundance of Light Elements
The abundance of light elements in the universe is a pivotal piece of evidence supporting the Big Bang theory. According to this theory, the universe began as an infinitely hot and dense point approximately 13.8 billion years ago. In its early stages, the universe was so hot that it formed a primordial soup of subatomic particles, including protons, neutrons, and electrons. As it expanded and cooled, these particles began to come together to form atomic nuclei—a process known as Big Bang nucleosynthesis (BBN). This period of nucleosynthesis lasted only a few minutes but was crucial in shaping the elemental composition of the universe. During BBN, protons and neutrons combined to form hydrogen (H), helium (He), lithium (Li), beryllium (Be), and boron (B). The abundance of these light elements is directly linked to the conditions during this brief window of nucleosynthesis. For instance, hydrogen and helium are by far the most abundant elements due to their simple structure and ease of formation under high-energy conditions. Helium-4 makes up about 24% of baryonic matter by mass, while hydrogen accounts for roughly 75%. Lithium-7 is present in much smaller quantities due to its more complex formation process. The observed abundances of these light elements closely match predictions derived from Big Bang theory. For example, if we look at distant stars or interstellar gas clouds that have not been significantly altered by stellar processes since their formation, we find that their compositions align with what would be expected from BBN calculations. This consistency provides strong evidence for the Big Bang model over alternative theories that do not predict such specific elemental abundances. Moreover, attempts to explain these abundances through other mechanisms have been unsuccessful. Stellar nucleosynthesis can create heavier elements but cannot account for the observed ratios of light elements like hydrogen and helium without invoking an initial state consistent with Big Bang conditions. The uniformity across different regions of space further supports a common origin rather than localized processes. In conclusion, the precise match between predicted and observed abundances of light elements serves as compelling cosmological evidence supporting the Big Bang theory. This alignment underscores our understanding that our universe began in an extremely hot state before expanding into its current form—a narrative supported by multiple lines of evidence including cosmic microwave background radiation and large-scale structure observations.
Large-scale Structure of the Universe
The large-scale structure of the universe is a compelling piece of cosmological evidence supporting the Big Bang theory. This structure refers to the distribution and organization of galaxies, galaxy clusters, and superclusters on vast scales. Observations reveal that these cosmic entities are not randomly scattered but instead form intricate networks and filaments, with vast voids in between. The Big Bang theory predicts that such structures evolved from tiny fluctuations in the universe's density during its early stages. These fluctuations, amplified by gravitational forces over billions of years, eventually led to the formation of galaxies and their subsequent clustering into larger structures. One key piece of evidence is the cosmic microwave background radiation (CMB), which shows minute variations in temperature across different regions. These variations are thought to be remnants from the early universe's density fluctuations. The CMB's patterns match predictions made by models based on the Big Bang theory, providing strong support for this cosmological framework. Furthermore, observations of galaxy distributions and redshift surveys have mapped out extensive networks of galaxy filaments and voids. These structures are consistent with simulations that model how matter would have evolved under gravitational forces since the Big Bang. For instance, projects like the Sloan Digital Sky Survey have mapped millions of galaxies across billions of light-years, revealing a web-like structure that aligns with theoretical expectations. Additionally, studies on gravitational lensing effects—where light from distant galaxies is bent by intervening mass concentrations—provide further insights into large-scale structure formation. This phenomenon helps trace out dark matter distributions that underpin visible galaxy clusters and superclusters. In summary, the large-scale structure of the universe offers robust evidence for the Big Bang theory through its consistent alignment with theoretical predictions about cosmic evolution from initial density fluctuations to current galactic distributions. This alignment underscores our understanding that our universe began as an infinitely hot and dense point before expanding into its current form over billions of years.
Modern Research and Future Directions
Modern research has revolutionized our understanding of the universe, propelling us toward unprecedented discoveries and future directions. Advances in telescopic technology have enabled scientists to peer deeper into the cosmos, uncovering secrets that were previously beyond our reach. Simulations and computational models have become indispensable tools, allowing researchers to predict and analyze complex phenomena with greater accuracy. These advancements have profound implications for understanding dark matter and dark energy, mysterious components that dominate the universe's mass-energy budget. As we continue to explore these frontiers, we are drawn closer to unraveling one of humanity's most enduring questions: The Origins of the Big Bang Theory.
Advances in Telescopic Technology
Advances in telescopic technology have revolutionized our understanding of the universe, enabling scientists to explore and study celestial phenomena with unprecedented precision. The development of next-generation telescopes, such as the James Webb Space Telescope (JWST) and the Square Kilometre Array (SKA), represents a significant leap forward in observational astronomy. These instruments are equipped with cutting-edge sensors and advanced optics that allow for deeper penetration into space, capturing images and data from distant galaxies, stars, and planets in greater detail than ever before. The JWST, for instance, features a segmented primary mirror that provides unparalleled resolution in infrared wavelengths, enabling researchers to study the formation of the first stars and galaxies in the early universe. Similarly, the SKA will boast an enormous collecting area that allows it to detect faint signals from distant objects with extraordinary sensitivity. These advancements not only enhance our ability to observe distant events but also facilitate groundbreaking discoveries about cosmic evolution and the origins of life. Furthermore, advancements in digital signal processing and machine learning algorithms are enhancing data analysis capabilities, allowing scientists to extract more meaningful insights from vast datasets generated by these powerful telescopes. As technology continues to evolve, future directions include integrating artificial intelligence into telescope operations for real-time data analysis and predictive maintenance. Additionally, there is a growing interest in space-based telescopes that can avoid atmospheric interference entirely, offering even clearer views of the cosmos. These innovations collectively propel modern research forward by providing new windows into understanding the Big Bang Theory and its implications for our universe's history and future trajectory.
Simulations and Computational Models
Simulations and computational models have revolutionized the field of modern research, particularly in understanding the Big Bang Theory. These tools allow scientists to recreate and analyze complex phenomena that are difficult or impossible to study directly. By leveraging advanced algorithms and powerful computing resources, researchers can simulate the early universe's evolution, including the formation of galaxies, stars, and planets. Computational models also enable the prediction of cosmic microwave background radiation patterns, which provide crucial evidence for the Big Bang Theory. Additionally, simulations help in understanding dark matter and dark energy's roles in shaping the universe's structure and expansion. These advancements not only deepen our understanding of cosmic history but also guide future research directions by identifying key areas for experimental verification and theoretical refinement. As technology continues to improve, simulations will become even more sophisticated, offering unprecedented insights into the mysteries of the cosmos.
Implications for Understanding Dark Matter and Dark Energy
The Big Bang Theory, a popular sitcom that aired from 2007 to 2019, offers a unique blend of humor and science. For those interested in watching this show, there are several platforms where it can be streamed. The series is available on major streaming services such as Netflix, Hulu, and Amazon Prime Video. Additionally, episodes can be purchased or rented through digital stores like iTunes and Google Play. Cable television networks often air reruns of the show as well. For those who prefer DVDs, complete seasons and box sets are available for purchase online through retailers like Amazon or in-store at local media outlets. Whether you're a fan of Sheldon Cooper's eccentricities or Howard Wolowitz's space adventures, there are numerous ways to enjoy The Big Bang Theory from the comfort of your home.