Weather Where I Am
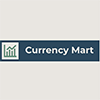
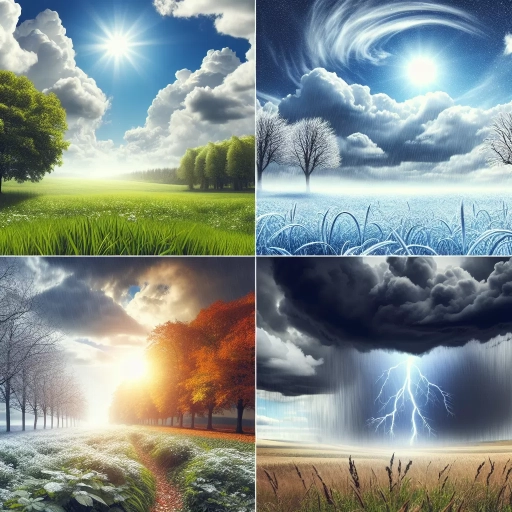
Weather is an integral part of our daily lives, influencing everything from our mood and activities to the economy and environment. Understanding weather patterns is crucial for predicting and preparing for various climatic conditions. This article delves into the intricacies of weather, exploring how it shapes our daily routines and impacts our lives in profound ways. We will also examine the technological advancements that have revolutionized weather monitoring, enabling us to forecast with greater accuracy than ever before. By grasping these aspects, we can better appreciate the complex dynamics of weather and its multifaceted influence on our world. Let's begin by understanding weather patterns, which form the foundation of this comprehensive exploration.
Understanding Weather Patterns
Understanding weather patterns is a complex and multifaceted field that encompasses various aspects of atmospheric science. At its core, it involves the study of global atmospheric circulation, which includes the movement of air masses and the formation of high and low-pressure systems. This foundational knowledge helps explain why certain regions experience specific types of weather throughout the year. Additionally, seasonal changes and climate zones play a crucial role in shaping local weather conditions, influencing everything from temperature fluctuations to precipitation patterns. Furthermore, advancements in weather forecasting techniques have significantly improved our ability to predict weather events with greater accuracy. By delving into these interconnected elements—global atmospheric circulation, seasonal changes and climate zones, and weather forecasting techniques—we can gain a deeper understanding of weather patterns.
Global Atmospheric Circulation
Global Atmospheric Circulation is a fundamental concept in understanding weather patterns, as it describes the large-scale movement of air in the Earth's atmosphere. This circulation is driven by the uneven heating of the Earth's surface by the sun, which creates temperature gradients that influence wind patterns. The primary driver of atmospheric circulation is the difference in solar radiation received between the equator and the poles. Near the equator, intense sunlight warms the air, causing it to rise and create low-pressure areas. Conversely, at higher latitudes, cooler temperatures result in sinking air and high-pressure zones. The rising warm air near the equator moves towards the poles along with its heat content, creating a global conveyor belt of air masses. This movement is interrupted by Earth's rotation, leading to deflection of winds to the right in the Northern Hemisphere and to the left in the Southern Hemisphere—a phenomenon known as Coriolis force. As a result, wind patterns form distinct circulation cells such as Hadley, Ferrel, and Rossby cells. Hadley cells are characterized by rising air near the equator that cools as it ascends and eventually sinks around 30 degrees latitude north and south. This sinking motion leads to dry desert regions like the Sahara Desert. Ferrel cells operate between 30 degrees latitude and 60 degrees latitude where winds are generally westerly (from west to east). Rossby waves or jet streams form at higher altitudes within these cells; they play a crucial role in shaping mid-latitude weather patterns by influencing storm tracks. Understanding Global Atmospheric Circulation helps meteorologists predict weather phenomena such as hurricanes, typhoons, monsoons, and even long-term climate trends like El Niño events. For instance, changes in atmospheric circulation can alter precipitation patterns significantly—monsoon rains rely on seasonal shifts in wind direction driven by differential heating between landmasses and oceans. In conclusion, Global Atmospheric Circulation underpins our comprehension of complex weather systems worldwide. By grasping how solar energy distribution drives air movement across different latitudes and altitudes—and how these movements interact with Earth's surface—we can better anticipate various weather events ranging from local storms to global climatic shifts. This knowledge not only aids scientific research but also informs practical applications like agriculture planning, aviation safety protocols, and disaster preparedness strategies essential for human well-being globally.
Seasonal Changes and Climate Zones
Seasonal changes and climate zones are intricately linked, influencing weather patterns across the globe. The Earth's tilt on its axis, approximately 23.5 degrees, is the primary driver of seasonal variations. As the planet orbits the sun, different parts of the Earth receive varying amounts of solar radiation throughout the year, resulting in distinct seasons—spring, summer, autumn (fall), and winter. These seasonal shifts not only affect temperature but also precipitation patterns and overall weather conditions. Climate zones, which are regions characterized by specific long-term weather conditions, play a crucial role in shaping these seasonal changes. The Earth is divided into several major climate zones: tropical, desert, temperate, polar, and alpine. Each zone has unique features that determine how seasons manifest locally. For instance: - **Tropical climates** near the equator experience minimal seasonal variation due to consistent sunlight exposure throughout the year. - **Desert climates** are known for extreme temperature fluctuations between day and night but generally remain hot during all seasons. - **Temperate climates** exhibit moderate seasonal changes with warm summers and cool winters. - **Polar climates** feature harsh winters with minimal sunlight during winter months and cool summers with extended daylight. - **Alpine climates**, found at high elevations regardless of latitude, often have cold temperatures year-round with significant snowfall. Understanding these climate zones is essential for predicting weather patterns because they influence local weather phenomena such as storms, droughts, and heatwaves. For example: - In temperate regions like North America or Europe, spring brings gentle rains while summer can be hot and humid; autumn (fall) is marked by cooling temperatures and colorful foliage; winter often brings snowfall. - In tropical regions like Southeast Asia or Central America, there may be a wet season followed by a dry season rather than traditional spring or autumn. The interplay between seasonal changes and climate zones also impacts global weather phenomena such as monsoons in India or typhoons in East Asia. Monsoons are seasonal wind shifts that bring heavy rainfall to certain regions during specific times of the year due to temperature differences between landmasses and oceans. In conclusion, recognizing how seasonal changes interact with various climate zones provides valuable insights into understanding broader weather patterns. This knowledge helps meteorologists predict future weather events more accurately while informing agricultural practices, urban planning initiatives, and environmental conservation efforts worldwide.
Weather Forecasting Techniques
Weather forecasting techniques have evolved significantly over the years, transforming from simple observations to sophisticated scientific methods. At the heart of modern weather forecasting lies a combination of traditional practices and cutting-edge technology. Meteorologists begin by gathering data from a vast network of weather stations, radar systems, and satellites orbiting the Earth. These tools provide real-time information on temperature, humidity, wind speed, atmospheric pressure, and precipitation patterns. Additionally, weather balloons and aircraft reconnaissance missions contribute valuable data from higher altitudes. One of the most critical techniques is numerical weather prediction (NWP), which involves using complex algorithms to analyze large datasets. These algorithms are run through powerful supercomputers that process millions of calculations per second to predict future weather conditions. The Global Forecast System (GFS) and European Centre for Medium-Range Weather Forecasts (ECMWF) models are among the most widely used NWP systems globally. Another essential technique is nowcasting, which focuses on predicting weather conditions over short periods—usually up to two hours. Nowcasting relies heavily on radar imagery and satellite data to track storms and other severe weather events in real-time. This method is particularly useful for issuing timely warnings for tornadoes, thunderstorms, and heavy rainfall. Doppler radar technology plays a crucial role in nowcasting by detecting wind shear and rotation within storms, helping meteorologists identify potential tornadoes early. Satellite imagery provides global coverage and helps track large-scale weather patterns such as hurricanes and typhoons. In recent years, advancements in artificial intelligence (AI) have also been integrated into weather forecasting techniques. AI models can analyze vast amounts of historical data to improve forecast accuracy by identifying patterns that may not be immediately apparent to human analysts. Furthermore, ensemble forecasting involves running multiple models with slightly different initial conditions to generate a range of possible outcomes. This approach helps quantify uncertainty in forecasts and provides a more comprehensive understanding of potential scenarios. Lastly, citizen science initiatives have become increasingly important as they leverage crowdsourced data from personal weather stations and mobile apps to enhance local forecast accuracy. In summary, modern weather forecasting combines traditional observation methods with advanced technological tools like NWP models, nowcasting techniques, Doppler radar systems, satellite imagery analysis, AI integration, ensemble forecasting approaches, and citizen science contributions. These integrated techniques enable meteorologists to provide highly accurate forecasts that help protect lives and property while supporting various industries such as agriculture, aviation, and emergency management.
The Impact of Weather on Daily Life
Weather plays a pivotal role in shaping our daily lives, influencing various aspects of our society and individual experiences. From the fields where crops grow to the roads we travel on, and from our health to emergency preparedness, weather's impact is multifaceted. Agriculture and food production are heavily dependent on weather conditions; droughts can lead to crop failures while excessive rains can cause flooding, both affecting food availability and economic stability. Transportation and infrastructure also face significant challenges due to weather; storms can disrupt air travel, heavy snowfall can paralyze road networks, and extreme temperatures can damage infrastructure. Additionally, weather has a direct impact on health; heatwaves can lead to heat-related illnesses, while cold snaps increase the risk of respiratory diseases. Understanding these impacts underscores the importance of emergency preparedness as communities must be ready to respond effectively during severe weather events. By delving into these areas—agriculture and food production, transportation and infrastructure, health—this article aims to provide a comprehensive overview of how weather shapes our daily lives. Transitioning seamlessly into understanding weather patterns will equip us with the knowledge needed to mitigate these effects and build more resilient communities.
Agriculture and Food Production
Agriculture and food production are intricately linked to the weather, as favorable climatic conditions are essential for crop growth and livestock health. Weather patterns such as temperature, precipitation, and sunlight exposure directly influence agricultural productivity. For instance, droughts can lead to crop failures and reduced yields, while excessive rainfall can cause flooding that damages crops and disrupts farming activities. Similarly, extreme temperatures can affect the quality of produce and the timing of harvests. Farmers often rely on weather forecasts to make informed decisions about planting, irrigation, and pest management. Advances in agricultural technology have also enabled more precise monitoring of weather conditions through satellite imaging and soil sensors, allowing for better crop management strategies. Moreover, weather impacts not only the quantity but also the quality of food produced. Climate change has been linked to shifts in growing seasons and increased frequency of extreme weather events like hurricanes and wildfires that can devastate agricultural lands. This variability poses significant challenges for food security globally. In regions where agriculture is a primary source of income, adverse weather conditions can lead to economic instability for farmers and their communities. The impact of weather on agriculture extends beyond immediate effects on crops; it also influences broader ecosystems that support biodiversity. Changes in precipitation patterns can alter soil moisture levels, affecting nutrient availability for plants and microbial activity in soil. Additionally, weather conditions influence pest populations and disease outbreaks among crops, necessitating adaptive strategies from farmers to maintain healthy agricultural ecosystems. In response to these challenges, sustainable agricultural practices such as agroforestry and conservation agriculture have gained prominence. These methods aim to enhance soil resilience against extreme weather events by promoting soil health through cover cropping and reduced tillage practices. Furthermore, integrating climate-resilient crop varieties into farming systems helps mitigate risks associated with unpredictable weather patterns. Overall, understanding the intricate relationship between agriculture and weather is crucial for ensuring global food security amidst increasing climatic uncertainties. By leveraging advanced technologies and adopting sustainable farming practices tailored to local climatic conditions, we can better adapt to changing weather patterns while maintaining productive agricultural systems that support human well-being worldwide.
Transportation and Infrastructure
Transportation and infrastructure play a crucial role in daily life, serving as the backbone of modern society. Efficient transportation systems ensure the smooth movement of people, goods, and services, while robust infrastructure supports economic growth and quality of life. However, weather conditions significantly impact these systems. Inclement weather such as heavy rain, snowstorms, or extreme heat can disrupt traffic flow by causing road closures, flight delays, and public transit cancellations. For instance, a severe snowstorm can paralyze urban areas by blocking roads and highways, leading to increased travel times and potential accidents. Similarly, flooding from heavy rainfall can damage road surfaces and bridges, necessitating costly repairs that divert resources from other essential projects. Moreover, weather-related disruptions have broader economic implications. Supply chains are often affected when transportation networks are compromised by adverse weather conditions. Delays in the delivery of goods can lead to stock shortages in retail stores and increased costs for businesses due to lost productivity and emergency measures. Additionally, extreme weather events like hurricanes or tornadoes can cause extensive damage to critical infrastructure such as power grids, water treatment plants, and communication networks. The aftermath of such events often requires significant investment in reconstruction efforts. In response to these challenges posed by weather on transportation and infrastructure systems, there is an increasing focus on resilience and adaptability. Modern technologies such as intelligent transportation systems (ITS) are being integrated into urban planning to enhance real-time monitoring and management of traffic flow during adverse weather conditions. ITS can reroute traffic dynamically based on current road conditions or provide early warnings for potential hazards like black ice or flash floods. Furthermore, sustainable infrastructure design is becoming more prevalent with an emphasis on climate-resilient materials and construction techniques that can withstand extreme weather events better than traditional methods. Green infrastructure solutions like permeable pavements help mitigate urban flooding by allowing rainwater to percolate through the surface rather than accumulating on it. In conclusion, the impact of weather on transportation and infrastructure is multifaceted—ranging from immediate disruptions in daily commutes to long-term economic repercussions due to damaged infrastructure. As our understanding of climate change evolves so does our approach towards building resilient transportation networks capable of withstanding various environmental challenges effectively while ensuring continued economic growth and improved quality of life for communities worldwide.
Health and Emergency Preparedness
Health and Emergency Preparedness is a crucial aspect of daily life, especially when considering the impact of weather. Extreme weather conditions such as hurricanes, floods, and heatwaves can pose significant threats to public health. For instance, hurricanes can cause widespread destruction leading to injuries and displacement, while floods can contaminate water sources and spread diseases. Heatwaves are known to exacerbate respiratory issues like asthma and can lead to heat-related illnesses such as dehydration and heatstroke. To mitigate these risks, communities must invest in robust emergency preparedness measures. This includes establishing early warning systems that alert residents of impending severe weather events. Public education campaigns are also essential; they inform people about the dangers associated with different types of weather phenomena and provide practical tips on how to stay safe during emergencies. For example, knowing how to stock an emergency kit with essentials like first aid supplies, non-perishable food items, and clean water can be lifesaving. Moreover, healthcare infrastructure plays a vital role in emergency preparedness. Hospitals and clinics should have contingency plans in place for managing large influxes of patients during natural disasters. This includes having backup power systems, stockpiling medical supplies, and training staff in disaster response protocols. Individuals also have a responsibility in health and emergency preparedness. They should stay informed about weather forecasts through reliable sources such as meteorological websites or mobile apps. Creating personal emergency plans that include evacuation routes and safe shelters is equally important. Additionally, maintaining good physical health through regular exercise and balanced diets helps build resilience against weather-related health challenges. In conclusion, health and emergency preparedness are integral components of coping with the impact of weather on daily life. By combining community-level initiatives with individual actions, we can significantly reduce the risks posed by extreme weather events. This holistic approach ensures that we are better equipped not only to survive but also thrive amidst unpredictable climatic conditions.
Technological Advances in Weather Monitoring
Technological advances in weather monitoring have revolutionized our ability to predict and understand weather patterns. The integration of satellite imaging and radar systems provides high-resolution data on atmospheric conditions, enabling precise tracking of storms and other weather phenomena. Weather stations, equipped with sophisticated sensors, collect detailed information on temperature, humidity, wind speed, and other meteorological factors. Additionally, artificial intelligence (AI) is being increasingly utilized in forecasting to analyze vast amounts of data quickly and accurately, improving the reliability of weather predictions. These technological advancements collectively enhance our understanding of weather patterns, allowing for better decision-making in various sectors such as agriculture, aviation, and emergency management.
Satellite Imaging and Radar Systems
Satellite imaging and radar systems have revolutionized weather monitoring by providing high-resolution data and real-time updates, significantly enhancing our ability to predict and respond to weather events. These technological advancements allow for the continuous observation of atmospheric conditions from space, enabling meteorologists to track storms, monitor temperature changes, and detect early signs of severe weather phenomena. Satellite imaging systems utilize various spectral bands to capture detailed images of cloud patterns, precipitation, and other atmospheric features. For instance, geostationary satellites like GOES-16 in North America provide full-disk images every 10 minutes, offering unparalleled temporal resolution for tracking rapid changes in weather systems. Radar systems complement satellite data by providing precise information on precipitation intensity and movement at ground level. Doppler radar technology can detect wind shear and rotation within storms, crucial for predicting tornadoes and other dangerous weather events. Phased array radar systems further enhance this capability with their ability to steer beams electronically without moving parts, allowing for faster scanning times and more accurate data collection. The integration of satellite imaging with radar data creates a comprehensive view of weather patterns at multiple scales—from global trends observed from space down to localized conditions detected by ground-based radar. This synergy supports more accurate forecasting models that can predict weather events hours or even days in advance. Additionally, advancements in data processing algorithms enable quicker analysis of vast amounts of data from these systems, facilitating timely warnings that save lives during natural disasters. Moreover, satellite imaging plays a critical role in monitoring long-term climate changes such as sea ice coverage, deforestation rates, and ocean currents—all indicators of broader environmental shifts. By combining historical satellite imagery with current observations from radar systems, scientists can identify trends that inform policy decisions on climate change mitigation strategies. In conclusion, satellite imaging and radar systems represent a cornerstone of modern weather monitoring technology. Their combined capabilities ensure that meteorologists have access to comprehensive real-time data essential for accurate forecasting and effective disaster management. As these technologies continue to evolve—driven by innovations in sensor design and data analytics—they will remain indispensable tools for understanding our dynamic atmosphere and protecting communities worldwide from adverse weather conditions.
Weather Stations and Data Collection
Weather stations are pivotal in modern weather monitoring, serving as the backbone of data collection that fuels accurate forecasting and climate research. These stations, ranging from simple backyard setups to sophisticated professional installations, gather a wide array of meteorological data including temperature, humidity, wind speed, atmospheric pressure, and precipitation levels. The technological advancements in weather monitoring have significantly enhanced the capabilities of these stations. For instance, automated weather stations equipped with sensors and transmitters can relay real-time data to central databases or even directly to the internet for global access. This real-time data collection allows for more precise short-term forecasts and better understanding of long-term climate trends. Advanced weather stations also incorporate cutting-edge technologies such as radar systems and satellite imaging to provide comprehensive coverage. Radar systems use radio waves to detect precipitation and other weather phenomena over large areas, while satellite imaging offers a bird's-eye view of global weather patterns. These technologies complement ground-based measurements by providing spatial context and enabling meteorologists to track storms and other significant weather events across vast distances. Moreover, the integration of IoT (Internet of Things) technology has revolutionized how weather data is collected and disseminated. IoT-enabled sensors can be deployed in remote or hard-to-reach areas where traditional infrastructure may not be feasible. These sensors communicate wirelessly with central hubs or cloud platforms, ensuring continuous data flow even in challenging environments. Additionally, mobile apps connected to these networks allow users to access current conditions and forecasts on-the-go. The precision of modern weather stations is further enhanced by advancements in sensor technology. High-resolution sensors can detect minute changes in environmental conditions that were previously undetectable. This increased sensitivity not only improves forecast accuracy but also aids in early warning systems for severe weather events like tornadoes or hurricanes. Furthermore, big data analytics plays a crucial role in processing the vast amounts of information generated by these advanced weather stations. Sophisticated algorithms analyze historical trends alongside real-time inputs to predict future weather patterns with greater reliability than ever before. This integration of big data analytics with IoT capabilities ensures that meteorologists have access to actionable insights that can inform decision-making at both local and global levels. In conclusion, contemporary weather stations represent a synergy between traditional meteorological practices and cutting-edge technological innovations. By leveraging advanced sensors, IoT connectivity, radar systems, satellite imaging, and big data analytics, these stations provide unparalleled insights into our dynamic atmosphere—insights that are crucial for everything from daily planning to long-term climate policy development.
Artificial Intelligence in Forecasting
Artificial Intelligence (AI) has revolutionized the field of weather forecasting, significantly enhancing the accuracy and reliability of meteorological predictions. By leveraging advanced machine learning algorithms, AI systems can analyze vast amounts of data from various sources, including satellite imagery, radar systems, and ground-based weather stations. This comprehensive data integration allows for more precise modeling of atmospheric conditions and better anticipation of weather patterns. For instance, deep learning techniques such as neural networks can identify complex patterns in historical climate data that traditional methods might overlook, leading to improved short-term and long-term forecasts. Additionally, AI-driven models can quickly adapt to new data inputs in real-time, enabling rapid updates and adjustments to forecast models as weather conditions evolve. This adaptability is particularly crucial for predicting severe weather events like hurricanes or tornadoes where timely warnings are essential for public safety. Furthermore, AI's ability to process large datasets efficiently reduces the computational time required for generating forecasts, making it possible to provide frequent updates throughout the day. The integration of AI with other technological advancements in weather monitoring—such as drones equipped with sensors and IoT devices—further enriches the data pool available for analysis. Overall, the incorporation of Artificial Intelligence into forecasting systems represents a significant leap forward in meteorology by enhancing predictive capabilities while ensuring faster response times to changing weather conditions.